2022.07.02.1
Files > Volume 7 > Vol 7 No 2 2022

Control of vibriosis in shrimp through the management of the microbiota and the immune system

Mery Ramírez1,5, Alexis Debut3, Frank Alexis4, Jenny Rodríguez1,2,*
1 Escuela Superior Politécnica del Litoral, ESPOL, Centro Nacional de Investigaciones Marinas (CENAIM), Campus Gustavo Galindo Km. 30.5 Vía Perimetral, P.O. Box 09-01-5863, Guayaquil, Ecuador
2 Faculty of Life Sciences (FCV), Escuela Superior Politécnica del Litoral, ESPOL, Ecuador
3 Universidad de las Fuerzas Armadas ESPE, Centro de Nanociencia y Nanotecnología, Avenida General Rumiñahui S/N y Ambato, P.O. Box 171-5-231B, Sangolquí, Ecuador.
4 Universidad San Francisco de Quito USFQ, Politécnico, Quito 170901, Ecuador.
5 Universidad Estatal Península de Santa Elena UPSE, Avda. principal La Libertad- Santa Elena, Ecuador.
* Corresponding author: [email protected] (Jenny Rodríguez)
Work phone number: +593 (04) 3035099. Tel.: +353-(0)993069228
Available from: http://dx.doi.org/10.21931/RB/2022.07.02.1
ABSTRACT
Shrimp aquaculture is constantly threatened by recurrent outbreaks of diseases caused by pathogenic bacteria of the genus Vibrio. Acute hepatopancreatic necrosis disease (AHPND) is one of the most aggressive vibriosis reported to date in the shrimp industry. AHPND provokes massive mortalities, causing economic losses with strong social impacts. Control of vibriosis requires the application of multifactorial strategies. This includes vibrio exclusion, shrimp microbiota, particularly in the digestive tract, and shrimp health management through immune stimulation. This paper reviews these two strategies for the prophylactic control of vibriosis. First, we describe the devastating effects of AHPND and the cellular and humoral effectors of the shrimp immune system to cope with this pathology. Secondly, the mechanisms of action of probiotics and their positive impacts are highlighted, including their immunostimulant effects and their role in the balance of the shrimp microbiota. Finally, we reviewed immunostimulants and prebiotics polysaccharides that together with probiotics act benefiting growth, feed efficiency and the microbiota of the digestive tract of farmed shrimp.
Keywords: Penaeus vannamei, Vibrios, probiotics, immunostimulant, prebiotics.
INTRODUCTION
Penaeus vannamei shrimp aquaculture is one of the fastest-growing aquaculture sectors worldwide1. In Latin America, the productions of Ecuador, Mexico, Honduras and Brazil stand out2. However, outbreaks of infectious diseases caused by pathogenic agents such as viruses and bacteria3–5 limit the industrial development of shrimp farming. Among the most aggressive viral diseases that have attacked farmed shrimp are white spot syndrome (WSSV)6–8 and Taura syndrome (TSV)9,10. Unlike viruses, bacteria, can remain indefinitely in culture systems because of their ability to produce biofilms5. Vibrios spp are the most aggressive bacteria for farmed shrimp, causing recurrent and emerging diseases11–13.
Strains of Vibrio parahaemolyticus and other vibrio species carrying the toxin, PirA and PirB are highly virulent for penaeid shrimp14–16 because they can cause acute hepatopancreatic necrosis disease (AHPND)17–19. AHPND causes significant losses in the shrimp industry globally and socioeconomic impacts20 that are estimated at more than $1 billion per year21. V. parahaemolyticus is a marine microorganism, which can grow in estuarine waters with tolerance to high temperature, high pH and high salinities with an affinity for marine plankton22,23. The success of vibrios is based on their ability to grow in shrimp culture systems characterized by high temperatures, high salinity 24 abundant presence of exuviae and high organic matter load. Under these conditions, vibrios thrive and modulate the microbiome at their advantage25.
Among the aspects that should be considered in closed systems designed to prevent the entry of vibrios and other potential pathogens are adequate nutrition with functional additives to improve the immune response26,27 and biosecurity controls28. In semi-extensive cultures, which involve earthen ponds in estuarine environments biosecurity is not sufficient and other alternatives must be considered to protect shrimp against vibriosis28. Among these alternatives, there are anti-virulence therapy 29,30, probiotics31,32 and immunomodulation33,34.
The main objective of probiotics is to exclude pathogenic bacteria from culture systems. In addition, probiotics enhance host growth and health by improving stress resistance 35,36 and promoting better nutrient utilization in shrimp by improving feed digestibility 37,38. Despite their effectiveness, the application of living organisms faces several challenges due to the preparation, bioactivity, stability, and success in different aquaculture environments. Another promising strategy is the enhancing of immune defenses. Immuno-stimulation seeks to activate the shrimp immune system based on the use of pathogen-associated molecular patterns (PAMPs) or molecules that resemble them and the recognition by their receptors in the host39,40, thus increasing immune resistance. Different polysaccharides fall in this category, among these, β-glucans and peptidoglycans41. The synergistic use of probiotics and immunostimulants 26,42 or the use of polysaccharides as prebiotics that help the establishment and aptitude of probiotics27,43 are also explored to face vibriosis. In this review, we present the devastating effects of AHPND and the cellular and humoral effectors of the shrimp immune system to cope with this pathology. We also report the strategies to control vibriosis in farmed shrimp, based on probiotics and immunostimulants.
Vibriosis in shrimp farming focusing on AHPND
Diseases produced by species of the genus Vibrio have caused significant economic losses in the shrimp industry worldwide20,44. Belonging to the phylum Proteobacteria, class Gammaproteobacteria, the genus Vibrio of the family Vibrionaceae includes 65 well-documented species of heterotrophic bacteria45. Vibrio harveyi and V. parahaemolyticus belonging to the clade Vibrio harveyi are among the most critical pathogens for shrimp46. Vibriosis cause massive mortality rates and can affect 100% of production8,12,47. Vibrios cause tail necrosis, loose shell syndrome, white gut, septic hepatopancreatis, systemic and wound vibriosis, causing hypoxia and death of shrimp from hatchery tanks to growing ponds12.
AHPND, the most aggressive vibriosis recorded to date, was detected in China in 2009 and other Southeast Asian countries48. In 2013, this disease was reported in Mexico 18 and spread to South America 49,50. PirA and PirB toxin genes were detected in other vibrio species of the V. harveyi clade51, Vibrio owensii52, Vibrio Campbelli 53 and recently in a Vibrio not belonging to this clade, Vibrio punensis50. The observed damage to shrimp health by AHPND has been devastating. Vibrios initiate their attack by colonizing the stomach14 of infected shrimp, originating dysbiosis 25,54 and generating toxins that subsequently cause hepatopancreatic cell damage13 and eventually hepatopancreatic septicemia 5,17. Shrimp affected by AHPND show severe necrosis in the hepatopancreas with massive detachment of epithelial cells and total loss of tissue structure. Pale atrophied hepatopancreas, intestinal epithelial necrosis and massive infiltration of hemocytes are observed55. It has also been reported that V. parahaemolyticus strains carrying PirA and PirB toxin genes alter the microbiome of infected shrimp, decreasing bacterial diversity and increasing the fitness of vibrios to proliferate in culture environments25,56.
Shrimp immune system
Shrimp, like all crustaceans, possess an immune system that effectively protects them from harmful microorganisms57. In penaeid shrimp, two lines of immune defense can be distinguished. The first line is a physical barrier formed by the cuticle which is mainly composed of polysaccharides, chitin, and mineral deposits58. Internally, contact with the medium is very limited due to the presence of cuticle in the stomach59, the constantly growing peritrophic membrane lining the epithelium of the midgut and hindgut 60 and the gastric sieve that limits passage into the hepatopancreas to particles smaller than 1 μm. The second line of defense is its innate immune system. It is based on humoral and cellular effectors, being hemocytes the main effectors of immunity. These effectors act together to eliminate infectious microorganisms61.
Innate immunity with specificity or immune priming has also been described in shrimp and other arthropods. This is based on the Down syndrome cell adhesion molecule (Dscam), a hypervariable recognition protein produced by alternative splicing. Dscam may be involved in both innate immunity and pathogen-specific immune response62. Shrimp cells innately recognize invading microbes through pattern-recognition proteins (PRRs) that act as pathogen-associated molecular pattern receptors (PAMPs)63. Innate immune cells respond rapidly to this activation through intracellular signaling cascades leading to humoral and cellular immunity activation 64.
Humoral mechanisms
Humoral effectors involve the synthesis and secretion of immune proteins from hemocytes into the circulatory system or hemocele65 and the release of enzymes of the prophenoloxidase (proPO) cascade leading to the generation of oxidative metabolites and deposition of toxic melanin66. Pathogens are recognized by pattern recognition proteins that recognize lipopolysaccharide (LPS) and β-1,3-glucan (BGBP)67–69, initiating the chain of proPO activation, culminating in melanization of the wound or intruding organisms70. Another mechanism of humoral response is the clotting system. The clotting cascade involves both hemocytes, which release transglutaminase71, and the coagulation factor present in the plasma72. Released transglutaminase polymerize the plasmatic clotting factor72.
Upon microbial challenge, hemocytes release antimicrobial peptides (AMPs)73. AMPs are cationic or anionic proteins that attack invading microorganisms 65,74. AMPs are small, from 15 to 100 amino acids and amphipathic in structure75. They can rapidly diffuse to the point of infection and destabilize the membrane of microorganisms, acting as antibiotics against gram-negative and gram-positive bacteria76 and against yeasts and filamentous fungi77. It has also been reported that AMPs modulate immunity74,78. In Crustacean the main AMPs are represented by the three families of cationic peptides: penaeidins, crustins and antilipopolysaccharide factor (ALF)79,80. Shrimp AMPs can be classified as cationic and anionic, the former being more abundant. Only stylicin, a peptide derived from hemocyanin and some ALFs are anionic AMPs65. Each family of shrimp AMPs shows sequence diversity and constitute multiple classes, isoforms, or subgroups75.
Penaeidins have affinity for shrimp chitin, being detected in the cuticle after microbial challenge73,81. Penaeidin mRNAs are present in all larval stages from nauplius onwards but vary in expression levels82. In penaeid shrimp, crustin homologs have been primarily identified by expressed sequence tag (EST) analysis of cDNA libraries from hemocytes of P. vannamei, P. setiferus83, and P. monodon84,85. Crustin is the largest AMP family reported in crustaceans86. In shrimp, most crustins are constitutively expressed in hemocytes but not in the hepatopancreas80,87,88. Some P. monodon crustin isoforms are expressed primarily in tissues other than hemocytes. ALFs are 10 to 14 kDa molecules. In shrimp, they were first identified in hemocytes of P. setiferus87 and P. monodon84,89. ALFs exhibit a potent minimum inhibitory concentration (MIC) (<6.25 μM) and spectrum of antimicrobial activity, covering a large number of gram-positive and gram-negative bacteria, filamentous fungi and even enveloped viruses90,91.
Cellular mechanisms
Cellular response includes all those reactions directly mediated by hemocytes in processes such as phagocytosis92, and nodule formation93. The classification and identification of hemocytes is based on morphological, antigenic, and functional aspects93,94. The three types of hemocytes are: (1) hyaline hemocytes which do not possess granules, which adhere and spread quickly, have phagocytic capacity and are in charge of clotting95; (2) small granular hemocytes possess abundant small granule, (Figure 1A) are involved in phagocytosis, encapsulation and melanization and synthesize and release AMPs; (3) large granular hemocytes (Figure 1A), which are loaded with large granules that store recognition proteins, adhesion proteins, regulatory enzymes, AMPs81 and proPO system96.
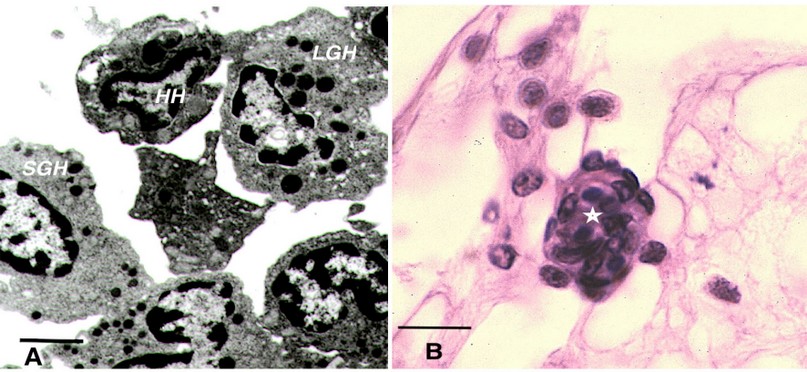
Figure 1. Shrimp hemocytes. (A) Transmission electron micrograph of shrimp hemocytes, bar = 2 m. (B) H&E staining of hemocytic nodule (star) in connective tissue of shrimp, bar 12 m. HH: Hyaline hemocytes, SGH: Small granular hemocytes, LGH: Large granular hemocytes.
A lower-than-normal number of circulating hemocytes in crustaceans correlates with reduced resistance to pathogens97, while an increase in the number of hemocytes enhances the immune response during periods of stress, increasing resistance to diseases 98,99. In addition, wound repair processes in shrimp are influenced by the concentration of hemocytes and penaeidins released by them in the wound area to eliminate invasive pathogens and improve hemocyte adhesion100. The open circulatory system of shrimp101 allows hemocytes to infiltrate and adhere to many tissues through the interstitial spaces of different organs and tissues without being limited to the vascular system102.
Hemocytic nodules, detected in connective tissue (Figure 1B), gills and hepatopancreas, are formed by numerous hemocytes that act synergistically to trap microorganisms or large antigens that cannot be eliminated by phagocytosis. These nodules undergo subsequent activation of the proPO system, with consequent melanization and destruction of microbes103 , such as the hemocytic nodules observed in tubules of the hepatopancreas of shrimp infected by vibrios18,104. If the lesions are very large, they will form hemocytic capsules like those observed around hepatopancreatic tubules injured by vibrios105. In shrimp, typical inflammatory response reactions with hemocyte infiltration and melanin deposition in nodules and capsules are observed during bacterial infections106 (Figure 2A). The respiratory burst, the mechanism that leads to the generation of reactive oxygen intermediates, is another microbicidal process used by shrimp hemocytes in phagocytosis and encapsulation107
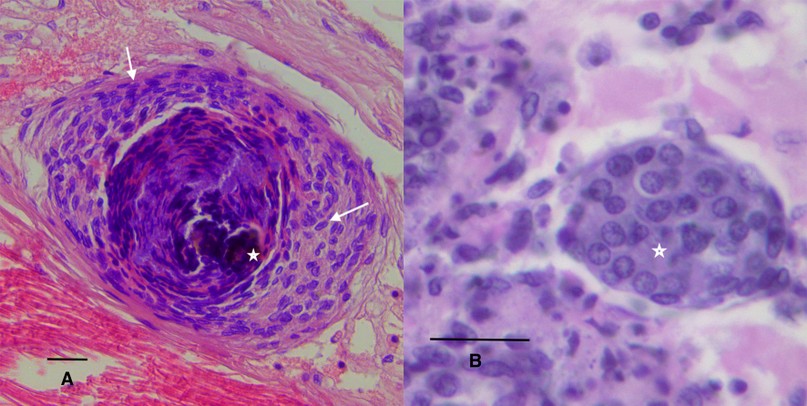
Other immune tissues
In penaeids shrimp, some of the defense functions are performed in the lymphoid organ (LO), which actively participates in capturing, isolating, and eliminating pathogens by phagocytosis108,109. The LO is a bilobed structure located in the anteroventral region of the hepatopancreas and posterior to the antennal gland, being an integral part of the distal portion of the subgastric artery110,111. The LO is considered an integral part of the circulatory system of penaeid shrimp and is believed to work as a hemolymph filter110,112. In histological sections it is seen as a vascularized tissue surrounded by hemal sinuses. The LO tubules have an inner layer of endothelial cells, surrounded by stromal tissue, bound by connective tissue109,110,113. In the presence of natural or experimental infections (mainly of viral etiology), nodular hyperplasia known as lymphoid organ spheroids (LOS) form (Figure 2B). These structures have also been detected in the connective tissues of other organs of shrimp and are called ectopic spheroids114,115. It has been reported that the LO filters viral particles of TSV114 and WSSV116. In late stages of infection, viral particles are detected in the spheroids of the lymphoid organ, whose cells enter apoptosis114,116. In addition to LOS, apoptosis has been reported in viral infections as a control mechanism of viral replication114,116. Other tissues involved in hemolymph clearance mechanisms are the heart reserve phagocytes, podocytes of the gills, and antennal gland117. The antennal gland is an important excretory organ in shrimp that is functionally similar to the kidney in vertebrates118,119, and therefore exposed to external medium.
Prophylactic methods to control vibriosis in shrimp culture
Vibriosis is the leading bacterial disease-causing mortality of cultured shrimp120,31. Antibiotics and chemotherapeutic agents were the first tools to control vibriosis. However, antibiotics have led to the development of resistant microorganisms121 with risk to human health due to the horizontal transfer of resistance genes from shrimp vibrios to human pathogenic bacteria122,123. The use of antibiotics also carries risks to the environment, due to the accumulation of environmental residues124. In addition, excessive levels of antibiotics can deteriorate the general condition of shrimp125 and cause rejection of the final product in international markets. Therefore, implementing effective and environmentally friendly multifactorial strategies for the control of vibrios in shrimp production systems is to be considered a priority126. These include the anti-virulence strategy127,128, which is based on blocking bacterial communication known as quorum sensing (QS), to inhibit the expression of virulence genes. The QS mechanism uses small chemical signaling molecules called autoinducers (AIs)129,130. This strategy has been applied in aquaculture and proposed by several authors as a promising tool for the control of pathogenic bacteria 128. Other alternatives to control vibriosis are the management of the microbiota, through probiotics 121,131,132 and immunostimulation31,133(Figure 3).
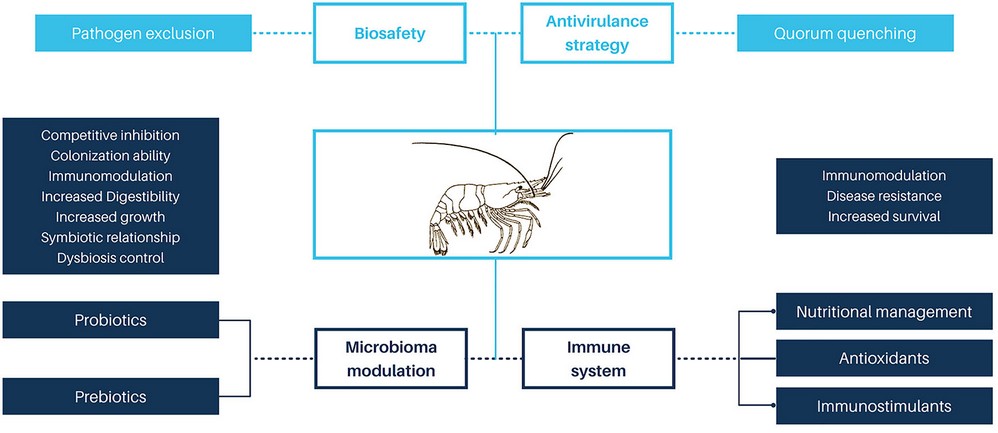
Figure 3. Approaches to control vibriosis in shrimp farming.
Probiotic
The term probiotic was defined by Fuller (1989)134 as "a live microbial food supplement that improves bacterial balance, benefiting the host's health”135. Probiotics emerge as a promising alternative to decrease the aquaculture industry's dependence on antibiotics 136. Probiotics have been applied in fish and invertebrate cultures137, especially in South America, Ecuador, and Asia, particularly China and India. Probiotics for aquaculture belong mainly to bacteria of the genera Bacillus, Lactobacillus. Pseudomonas, and vibrio, in addition to yeasts31,138,139.
In the field of aquaculture, the concept of probiotic is extended to “a live microbial supplement that has a beneficial effect on the host by improving the environmental microbial community, ensuring better use of the feed or its nutritional value, and improving the response to disease or the quality of culture environment”121. In addition probiotics can be applied directly to the culture water as bio remediators 140,141, the modes of action of probiotics are diverse142 (Table 1). Applied in feed, probiotics improve digestion, enhance immune response, and inhibit the growth of pathogenic bacteria143–145. Probiotics competitively exclude vibrios26,146,147 and enhance shrimp immune indicators, phagocytosis, nodule formation, activation of the proPO system and generation of AMPs148. Probiotics release several antibacterial agents with bactericidal or bacteriostatic properties that inhibit the colonization of pathogenic organisms in the shrimp gut149–151. Probiotics can modulate the expression of immune genes and gut microbiota during stress induced by high stocking density152.
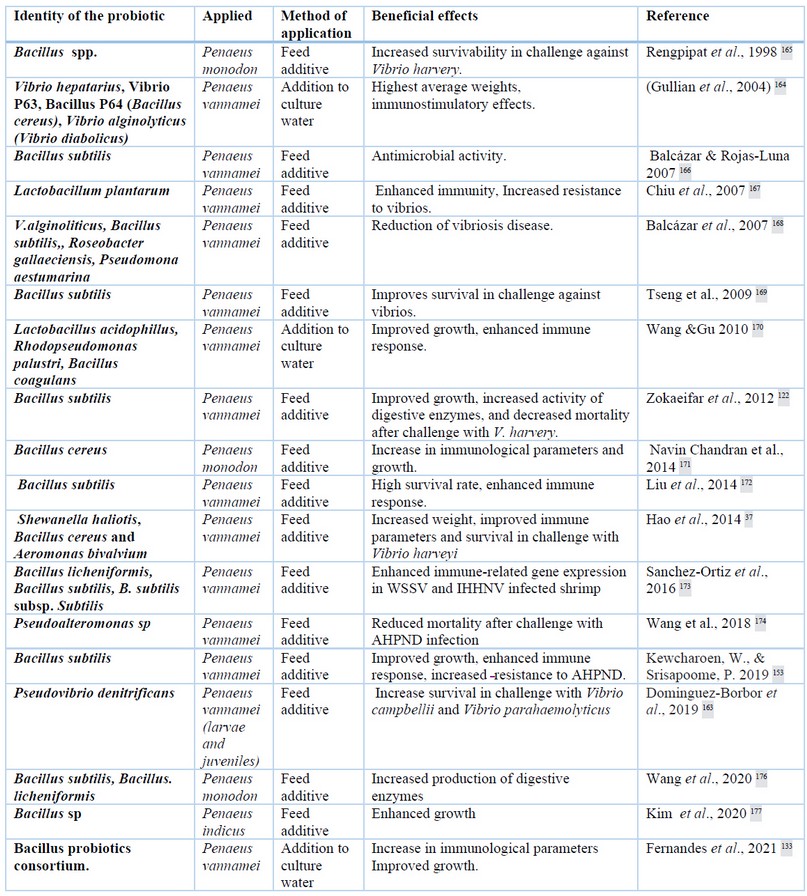
Table 1. Several probiotic bacteria applied in shrimp cultures
Bacteria of the Bacillus genus are the most widely used in shrimp aquaculture as probiotics because they form spores, which facilitates conservation and commercial distribution. In addition, Bacillus bacteria are well perceived by the consumer because they are gram-positive bacteria. Among other advantages of Bacillus spp are their ability to grow using different carbon and nitrogen sources153. Bacillus spp can grow in the marine environment and produce a variety of bioactive compounds that act on growth, health, disease resistance 154,155 and culture water quality156,157. It has been further reported that Bacillus spp. produce a wide range of antimicrobial peptides158,159. Bacillus spp. supplementation decreases mortality in shrimp exposed to pathogenic Vibrios160,161. The most used species are Bacillus subtilis and Bacillus lechiniformis. Other probiotics successfully used in aquaculture belong to other bacterial genera, including Vibrios162 or marine symbionts such as Pseudovibrio denitrificans163. Vibrio diabolicus is a very effective probiotic controlling vibriosis affecting P. Vannamei in larval stages164 and juveniles56. Because it is a vibrio, this bacterium has the advantage of thriving in the culture conditions of shrimp. V. diabolicus can compete effectively with pathogenic vibrios56, thus having positive effects on the immune system of shrimp133,164.
Considerations for the selection and use of probiotics in shrimp aquaculture
Several aspects must be considered for selecting and using probiotics in shrimp. Among these are the ability to grow in marine environments, exclude vibrios, and compete with them for colonization of shrimp and surfaces of culture systems to which vibrios are well adapted. Bacterial antagonism is a common phenomenon in nature; therefore, microbial interactions play an important role in balancing beneficial competitors and potentially pathogenic microorganisms 31. In this sense the ability to compete and colonize is one of the most desirable properties of probiotics. There are several aspects behind the ability to compete and colonize one of them is competitive exclusion, which occurs when an established microflora prevents or reduces the colonization of a bacterial competitor for the same location140. For pathogenic bacteria the ability to adhere to host substrates is related to virulence and is considered as the first step for an infection178.
Probiotics must have the ability to compete for mucosal attachment sites and competition for nutrients140. Therefore, the attachment ability of probiotics is another important criterion for their selection and application in aquaculture179,180. Colonization ability further depends on migration in a coordinated manner across organism surfaces181. For these processes, bacteria use cell appendages such as fimbriae and flagella, structures important for colonization and the creation of biofilms182–184. By producing inhibitory substances, probiotics prevent pathogen replication and reduce pathogen colonization132,140. Among the extracellular products that inhibit or kill other potentially pathogenic bacteria have been found: antimicrobial substances185,186, organic acids187, bacteriocins188, or hydrogen peroxide. Another important aspect is the morphophysiology of shrimp. Indeed, the shrimp has an external and internal cuticle covering the stomach, which is lost during molting, and the peritrophic membrane in continuous growth, covering the midgut and hindgut60. Therefore, probiotics with affinity for the cuticle and chitin, should be applied continuously.
New approaches have emerged to identify and isolate novel probiotics in recent years. One of them is the use of marine symbionts, which are perfectly adapted to marine environments. P. denitrificans is a symbiont that generates antimicrobial substances. It is metabolically versatile, exhibits competitive inhibition against several shrimp pathogenic Vibrio spp and increases survival against vibrio challenges 163. Another aspect that is gaining relevance is the ability of probiotics to recover or maintain healthy microbiota, curbing dysbiosis. The probiotic V. diabolicus can control the bacterial populations of the gastrointestinal tract of shrimp, restoring the microbial balance56.
Immuno-stimulation and Immunostimulants
The immune system can recognize and react to PAMPs through different PRRs189. Different PAMPs, such as LPS, peptidoglycans and β-glucans can activate the shrimp immune system, acting as immunostimulants. Immunostimulants are an effective alternative to prevent diseases in shrimp. There are several sources of these molecules in nature, including gram-negative bacteria (lipopolysaccharides), gram-positive bacteria (peptidoglycans), fungi, yeasts, and algae (β-glucans). The most used immunostimulants are β-glucans from different marine and terrestrial sources190. The β-glucans are polysaccharides other than starch; they are present in the cell wall of bacteria, fungi, and plants. They are used to increase the resistance of shrimp against bacterial and viral infections 26,191. The β-glucans extracted from different sources exhibit various immunomodulatory functions, acting as PAMPs, they interact with PRRs to activate the innate immune response192. In shrimp β-glucans extracted from yeast Candida parapsilosis has an immunomodulatory effect against WSSV, obtaining high survivals193. In addition to their effects on the immune system, β-glucans from bacteria improve the nutritional and antioxidative status of shrimp194. β-glucans from marine yeasts improve respiratory burst and phenoloxidase activity195. The beneficial effect of β-glucans can be enhanced by degradation to obtain oligo β-glucans with improvements in their immunostimulatory and growth, promoting effect in shrimp191. The benefits of using β-glucans have been evidenced by successful results in survival and performance in shrimp ponds26. Immunomodulatory effects on penaeid shrimp have been also reported for other polysaccharides that exhibit repeating molecular patterns, such as arabinose and galactose heteropolysaccharides from Angelica sinensis, and cocoa pectin196.
As previously described, innate immunity with specificity or immune priming has been reported in shrimp and other arthropods197. From the perspective of a possible pathogen-specific immune response, vaccination trials have been performed in shrimp. Thus in P. monodon the VP28 protein (WSSV) has been used as antigen, observing immunomodulatory effects on different immune effectors:caspase, penaeidin, crustin, astakine, syntenin, PmRACK, Rab7, STAT and C-type lectin198.
Prebiotics
Prebiotics are indigestible fibers for the host, but are digested by beneficial bacteria, which improve the health of the intestine199,200 and can be referred to as functional saccharides201. Moreover, prebiotics are associated with improvements in growth, feed efficiency, digestive enzyme activities, proper gut morphology, improved immune status and disease resistance136,202,203. Prebiotics such as fructooligosaccharides (FOS), galactooligosaccharide, mannan oligosaccharides, and inuline are applied in shrimp culture204. The application of short-chain fructooligosaccharides (ScFOs) in P. vannamei diet increased the weight and specific growth rate of shrimp205. ScFOS supplemented at concentrations of 0.025 to 0.800 wt% increase the respiratory burst hemocytes206. More details about prebiotics in farmed shrimp are shown in table 2.
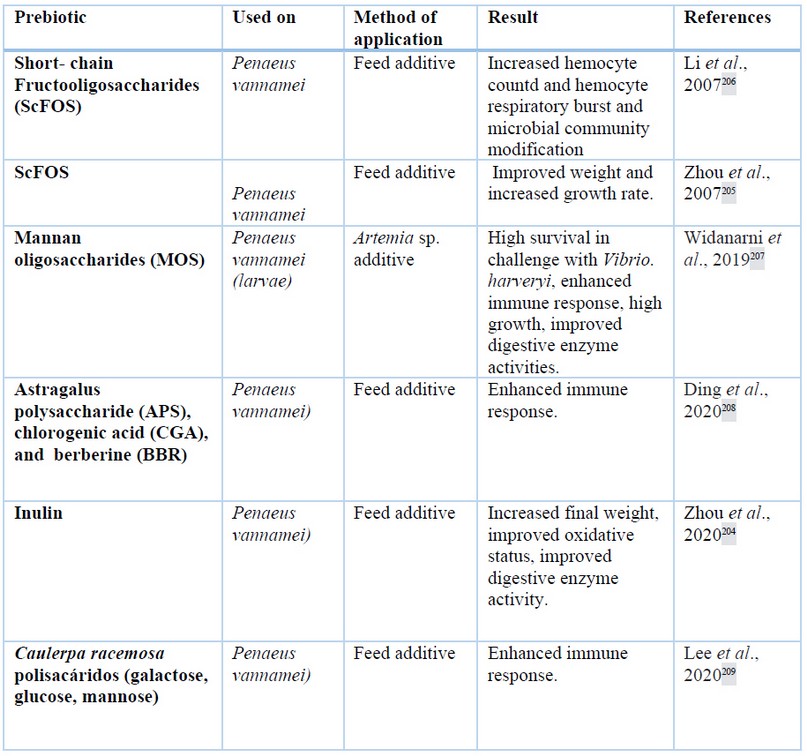
Table 2. Several prebiotic polysaccharides applied in shrimp cultures
CONCLUSION
The survival rate, yield, size, color, and taste of shrimps are critical for its market. With the increased loss of worldwide production due to vibriosis and the risks inherent in the use of antibiotics, new products to control vibriosis must consider the need for “organic” shrimps without antibiotics. Among the most promising alternatives are probiotics. The modes of action of probiotics are diverse. They improve digestion, enhance immune response, and inhibit the growth of pathogenic Vibrio spp. Probiotics release several antibacterial agents with bactericidal or bacteriostatic properties that inhibit the colonization of pathogenic Vibrio spp in the shrimp. During the last years, new approaches have emerged to identify and isolate novel probiotics. One of them is the use of marine symbionts, but even if first results are highly encouraging, a deeper understanding of the biological mechanisms is still needed. Another aspect that is gaining relevance is the ability of probiotics to recover or maintain healthy microbiota, curbing dysbiosis, and restoring microbial balance. The application of living organisms faces several challenges, due to their differences in bio activity, and effectiveness in marine aquaculture environments. Another promising option is the use of polysaccharides that increase shrimp resistance to pathogenic Vibrio spp. by immune stimulation. Polysaccharides can also act as prebiotics, being used as energy sources by beneficial bacteria, which improve the health of the host intestine. The significant advances in combating the AHPND in the shrimp industry have evidenced several strategies to solve this critical challenge, but it is not yet clear which is the best of the previously mentioned in this review. It is likely that while each has its advantages and disadvantages, a possible solution could be a combination of them.
REFERENCES
1. FAO. The State of Food Security and Nutrition in the World 2021. The State of Food Security and Nutrition in the World 2021 (2021).
2. Bondad-Reantaso, M. G., Subasinghe, R. P., Josupeit, H., Cai, J. & Zhou, X. The role of crustacean fisheries and aquaculture in global food security: Past, present and future. J. Invertebr. Pathol. 110, 158–165 (2012).
3. Mohney, L. L., Lightner, D. V. & Bell, T. A. An Epizootic of Vibriosis in Ecuadorian Pond‐Reared Penaeus vannamei Boone (Crustacea: Decapoda). J. World Aquac. Soc. 25, 116–125 (1994).
4. Noriega-Orozco, L., Acedo-Félix, E., Higuera-Ciapara, I., Jiménez-Flores, R. & Cano, R. Pathogenic and non pathogenic Vibrio species in aquaculture shrimp ponds. Rev. Latinoam. Microbiol. 49, 60–67 (2008).
5. Thitamadee, S. et al. Review of current disease threats for cultivated penaeid shrimp in Asia. Aquaculture 452, 69–87 (2016).
6. Chou Hsin-Yiu, Huang Chang-Yi, Wang Chung-Hsiung, Chiang Hsien-Choung & Lo Chu-Fang. Pathogenicity of a baculovirus infection causing white spot syndrome in cultured penaeid shrimp in Taiwan. Dis. Aquat. Organ. 23, 165–173 (1995).
7. Rodríguez, J. et al. White spot syndrome virus infection in cultured Penaeus vannamei (Boone) in Ecuador with emphasis on histopathology and ultrastructure. J. Fish Dis. 26, 439–450 (2003).
8. Flegel, T. W. Detection of major penaeid shrimp viruses in Asia, a historical perspective with emphasis on Thailand. Aquaculture 258, 1–33 (2006).
9. Hasson, K. W. et al. Taura syndrome in Penaeus vannamei: Demonstration of a viral etiology. Dis. Aquat. Organ. 23, 115–126 (1995).
10. Dhar, A. K. & Thomas Allnutt, F. C. Taura Syndrome Virus. Encycl. Virol. 1–8 (2008) doi:10.1016/B978-012374410-4.00782-2.
11. Lightner, D. V. & Redman, R. M. Shrimp diseases and current diagnostic methods. Aquaculture 164, 201–220 (1998).
12. Flegel, T. W. Historic emergence, impact and current status of shrimp pathogens in Asia. J. Invertebr. Pathol. 110, 166–173 (2012).
13. Tran, L. et al. Determination of the infectious nature of the agent of acute hepatopancreatic necrosis syndrome affecting penaeid shrimp. Dis. Aquat. Organ. 105, 45–55 (2013).
14. Lai, H. C. et al. Pathogenesis of acute hepatopancreatic necrosis disease (AHPND) in shrimp. Fish Shellfish Immunol. 47, 1006–1014 (2015).
15. Han, J. E., Tang, K. F. J., Aranguren, L. F. & Piamsomboon, P. Characterization and pathogenicity of acute hepatopancreatic necrosis disease natural mutants, pirABvp (‐) V. parahaemolyticus, and pirABvp (+) V. campbellii strains. Aquaculture 470, 84–90 (2017).
16. Xiao, J. et al. Shrimp AHPND-causing plasmids encoding the PirAB toxins as mediated by pirAB-Tn903 are prevalent in various Vibrio species. Sci. Rep. 7, 1–11 (2017).
17. Nunan, L., Lightner, D., Pantoja, C. & Gomez-Jimenez, S. Detection of acute hepatopancreatic necrosis disease (AHPND) in Mexico. Dis. Aquat. Organ. 111, 81–86 (2014).
18. Soto-Rodriguez, S. A., Gomez-Gil, B., Lozano-Olvera, R., Betancourt-Lozano, M. & Morales-Covarrubias, M. S. Field and experimental evidence of Vibrio parahaemolyticus as the causative agent of acute hepatopancreatic necrosis disease of cultured shrimp (LitoPenaeus vannamei) in northwestern Mexico. Appl. Environ. Microbiol. 81, 1689–1699 (2015).
19. Sirikharin, R. et al. Characterization and PCR detection of binary, pir-like toxins from vibrio parahaemolyticus isolates that cause acute hepatopancreatic necrosis disease (AHPND) in shrimp. PLoS One 10, 1–16 (2015).
20. Kua, B. C. et al. Current status of acute hepatopancreatic necrosis disease (AHPND) of farmed shrimp in Malaysia. Proceedlossesings ASEAN Reg. Tech. Consult. EMS/AHPND Other Transbound. Dis. Improv. Aquat. Anim. Heal. Southeast Asia 55–59 (2016).
21. FAO. Report of the FAO/MARD Technical Workshop on Early Mortality Syndrome (EMS) or Acute Hepatopancreatic Necrosis Syndrome (AHPNS) of Cultured Shrimp (under TCP/VIE/3304). FAO Fisheries and Aquaculture Report vol. 1053 (2013).
22. Wong, H. C. et al. Characterization of Vibrio parahaemolyticus isolates obtained from foodborne illness outbreaks during 1992 through 1995 in Taiwan. J. Food Prot. 63, 900–906 (2000).
23. Su, Y. C. & Liu, C. Vibrio parahaemolyticus: A concern of seafood safety. Food Microbiol. 24, 549–558 (2007).
24. Ponce-Palafox, J., Martinez-Palacios, C. A. & Ross, L. G. The effects of salinity and temperature on the growth and survival rates of juvenile white shrimp, Penaeus vannamei, Boone, 1931. Aquaculture 157, 107–115 (1997).
25. Chen, W. Y. et al. Microbiome Dynamics in a Shrimp Grow-out Pond with Possible Outbreak of Acute Hepatopancreatic Necrosis Disease. Sci. Rep. 7, 1–12 (2017).
26. Rodríguez, J. et al. Exposure to probiotics and β-1,3/1,6-glucans in larviculture modifies the immune response of Penaeus vannamei juveniles and both the survival to White Spot Syndrome Virus challenge and pond culture. Aquaculture 273, 405–415 (2007).
27. Zhang, J. et al. Effects of dietary mannan oligosaccharide on growth performance, gut morphology and stress tolerance of juvenile Pacific white shrimp, LitoPenaeus vannamei. Fish Shellfish Immunol. 33, 1027–1032 (2012).
28. Cornelio, C.-A. Manual de buenas prácticas de manejo para el cultivo de camaron blanco Penaeus vannamei. Organismo internafile:///C:/Users/Oscar Soriano/Documents/Buenas Prácticas Acuicolas/225254.pdfcional regional OIRSA . (2010).
29. Defoirdt, T., Boon, N., Sorgeloos, P., Verstraete, W. & Bossier, P. Quorum sensing and quorum quenching in Vibrio harveyi: Lessons learned from in vivo work. ISME J. 2, 19–26 (2008).
30. Rasko, D. A. & Sperandio, V. Anti-virulence strategies to combat bacteria-mediated disease. Nat. Rev. Drug Discov. 9, 117–128 (2010).
31. Balcázar, J. L. et al. The role of probiotics in aquaculture. Vet. Microbiol. 114, 173–186 (2006).
32. Farizky, H. S., Satyantini, W. H. & Nindarwi, D. D. The efficacy of probiotic with different storage to decrease the total organic matter, ammonia, and total Vibrio on shrimp pond water. IOP Conf. Ser. Earth Environ. Sci. 441, (2020).
33. Gullian, M. & Rodriguez, J. Immunostimulant qualities of probiotic bacteria. Glob. Aquacult 52–54 (2002).
34. Jun, J. W. et al. Phage Application for the Protection from Acute Hepatopancreatic Necrosis Disease (AHPND) in Penaeus vannamei. Indian J. Microbiol. 58, 114–117 (2018).
35. Liu, K. F., Chiu, C. H., Shiu, Y. L., Cheng, W. & Liu, C. H. Effects of the probiotic, Bacillus subtilis E20, on the survival, development, stress tolerance, and immune status of white shrimp, LitoPenaeus vannamei larvae. Fish Shellfish Immunol. 28, 837–844 (2010).
36. Wang, Y. C., Hu, S. Y., Chiu, C. S. & Liu, C. H. Multiple-strain probiotics appear to be more effective in improving the growth performance and health status of white shrimp, LitoPenaeus vannamei, than single probiotic strains. Fish Shellfish Immunol. 84, 1050–1058 (2019).
37. Hao, K. et al. Effects of dietary administration of Shewanella haliotis D4, Bacillus cereus D7 and Aeromonas bivalvium D15, single or combined, on the growth, innate immunity and disease resistance of shrimp, LitoPenaeus vannamei. Aquaculture 428–429, 141–149 (2014).
38. Olmos, J., Acosta, M., Mendoza, G. & Pitones, V. Bacillus subtilis, an ideal probiotic bacterium to shrimp and fish aquaculture that increase feed digestibility, prevent microbial diseases, and avoid water pollution. Arch. Microbiol. 202, 427–435 (2020).
39. Medzhitov, R. & Janeway, C. A. Innate immunity: The virtues of a nonclonal system of recognition. Cell 91, 295–298 (1997).
40. Janeway, C. A. & Medzhitov, R. Innate immune recognition. Annu. Rev. Immunol. 20, 197–216 (2002).
41. Amparyup, P., Sutthangkul, J., Charoensapsri, W. & Tassanakajon, A. Pattern recognition protein binds to lipopolysaccharide and β-1,3-glucan and activates shrimp prophenoloxidase system. J. Biol. Chem. 287, 10060–10069 (2012).
42. Al-Deriny, S. H. et al. The synergistic effects of Spirulina platensis and Bacillus amyloliquefaciens on the growth performance, intestinal histomorphology, and immune response of Nile tilapia (Oreochromis niloticus). Aquac. Reports 17, 100390 (2020).
43. Chen, M., Chen, X. Q., Tian, L. X., Liu, Y. J. & Niu, J. Beneficial impacts on growth, intestinal health, immune responses and ammonia resistance of pacific white shrimp (LitoPenaeus vannamei) fed dietary synbiotic (mannan oligosaccharide and Bacillus licheniformis). Aquac. Reports 17, 100408 (2020).
44. Prayitno, S. B. & Latchford, J. W. Experimental infections of crustaceans with luminous bacteria related to Photobacterium and Vibrio. Effect of salinity and pH on infectiosity. Aquaculture 132, 105–112 (1995).
45. Thompson, F., Brian, A. & Swing, J. The Biology of Vibrios. The Biology of Vibrios (ASM Press, 2006). doi:10.1128/9781555815714.
46. Zhou, J. et al. A nonluminescent and highly virulent Vibrio harveyi strain is associated with ‘bacterial white tail disease’ of LitoPenaeus vannamei shrimp. PLoS One 7, 19–22 (2012).
47. Lightner, D. V. & Ph, D. Early Mortality Syndrome Affects Shrimp In Asia. Glob. Aquac. Advocate 40, 2012 (2012).
48. Zorriehzahra, M. J. Early Mortality Syndrome (EMS) as new Emerging Threat in Shrimp Industry. Adv. Anim. Vet. Sci. 3, 64–72 (2015).
49. Saavedra-Olivos, K. Y. et al. Detection of a protein associated with acute hepatopancreatic necrosis disease (AHPND) in LitoPenaeus vannamei under semi-intensive farming in Ecuador. Rev. Investig. Vet. del Peru 29, 328–338 (2018).
50. Restrepo, L. et al. PirVP genes causing AHPND identified in a new Vibrio species (Vibrio punensis) within the commensal Orientalis clade. Sci. Rep. 8, 1–14 (2018).
51. Xiao, J. et al. Shrimp AHPND-causing plasmids encoding the PirAB toxins as mediated by pirAB-Tn903 are prevalent in various Vibrio species. Sci. Rep. 7, 1–11 (2017).
52. Liu, L. et al. A Vibrio owensii strain as the causative agent of AHPND in cultured shrimp, LitoPenaeus vannamei. J. Invertebr. Pathol. 153, 156–164 (2018).
53. Wangman, P. et al. PirA & B toxins discovered in archived shrimp pathogenic Vibrio campbellii isolated long before EMS/AHPND outbreaks. Aquaculture 497, 494–502 (2018).
54. Hossain, M. S., Dai, J. & Qiu, D. Dysbiosis of the shrimp (Penaeus monodon) gut microbiome with AHPND outbreaks revealed by 16S rRNA metagenomics analysis. Aquac. Res. 52, 3336–3349 (2021).
55. Joshi, J. et al. Variation in Vibrio parahaemolyticus isolates from a single Thai shrimp farm experiencing an outbreak of acute hepatopancreatic necrosis disease (AHPND). Aquaculture 428–429, 297–302 (2014).
56. Restrepo, L. et al. Microbial community characterization of shrimp survivors to AHPND challenge test treated with an effective shrimp probiotic (Vibrio diabolicus). Microbiome 9, 1–20 (2021).
57. Young Lee, S. & Soüderhaäll, K. Early events in crustacean innate immunity. Fish Shellfish Immunol. 12, 421–437 (2002).
58. Rowley, A. F. The Immune System of Crustaceans. Encyclopedia of Immunobiology vol. 1 (Elsevier, 2016).
59. Hackman, R. H. Chitin and the fine structure of cuticles. Chitin and Benzoylphenyl Ureas 1–32 (1987) doi:10.1007/978-94-009-4824-2_1.
60. Wang, P. & Granado, R. R. An intestinal mucin is the target substrate for a baculovirus enhancin. Proc. Natl. Acad. Sci. U. S. A. 94, 6977–6982 (1997).
61. Bachère, E. Anti-infectious immune effectors in marine invertebrates: Potential tools for disease control in larviculture. Aquaculture 227, 427–438 (2003).
62. Ng, T. H., Chiang, Y. A., Yeh, Y. C. & Wang, H. C. Review of Dscam-mediated immunity in shrimp and other arthropods. Dev. Comp. Immunol. 46, 129–138 (2014).
63. Cerenius, L., Lee, B. L. & Söderhäll, K. The proPO-system: pros and cons for its role in invertebrate immunity. Trends Immunol. 29, 263–271 (2008).
64. Tassanakajon, A., Somboonwiwat, K., Supungul, P. & Tang, S. Discovery of immune molecules and their crucial functions in shrimp immunity. Fish Shellfish Immunol. 34, 954–967 (2013).
65. Tassanakajon, A. et al. Shrimp humoral responses against pathogens: antimicrobial peptides and melanization. Dev. Comp. Immunol. 80, 81–93 (2018).
66. Hernández-López, J., Gollas-Galván, T. & Vargas-Albores, F. Activation of the prophenoloxidase system of the brown shrimp (Penaeus californiensis Holmes). Comp. Biochem. Physiol. - C Pharmacol. Toxicol. Endocrinol. 113, 61–66 (1996).
67. Sritunyalucksana, K. & Soderhall, K. The proPO and clotting system in crustaceans. Aquaculture 191, 53–69 (2000).
68. Vargas-Albores, F. & Yepiz-Plascencia, G. Beta glucan binding protein and its role in shrimp immune response. Aquaculture 191, 13–21 (2000).
69. Chen, Y. Y. et al. Lipopolysaccharide and β-1,3-glucan-binding protein (LGBP) bind to seaweed polysaccharides and activate the prophenoloxidase system in white shrimp LitoPenaeus vannamei. Dev. Comp. Immunol. 55, 144–151 (2016).
70. Cerenius, L. & Söderhäll, K. The prophenoloxidase-activating system in invertebrates. Immunol. Rev. 198, 116–126 (2004).
71. Maningas, M. B. B., Kondo, H., Hirono, I., Saito-Taki, T. & Aoki, T. Essential function of transglutaminase and clotting protein in shrimp immunity. Mol. Immunol. 45, 1269–1275 (2008).
72. Ratcliffe, N. A., Rowley, A. F., Fitzgerald, S. W. & Rhodes, C. P. Invertebrate Immunity: Basic Concepts and Recent Advances. Int. Rev. Cytol. 97, 183–350 (1985).
73. Muñoz, M., Vandenbulcke, F., Saulnier, D. & Bachère, E. Expression and distribution of penaeidin antimicrobial peptides are regulated by haemocyte reactions in microbial challenged shrimp. Eur. J. Biochem. 269, 2678–2689 (2002).
74. Brown, K. L. & Hancock, R. E. W. Cationic host defense (antimicrobial) peptides. Curr. Opin. Immunol. 18, 24–30 (2006).
75. Tassanakajon, A., Amparyup, P., Somboonwiwat, K. & Supungul, P. Cationic Antimicrobial Peptides in Penaeid Shrimp. Mar. Biotechnol. 13, 639–657 (2011).
76. Brogden, K. A. Antimicrobial peptides: Pore formers or metabolic inhibitors in bacteria? Nat. Rev. Microbiol. 3, 238–250 (2005).
77. Bandeira, P. T. et al. A Type IIa crustin from the pink shrimp Farfantepenaeus paulensis (crusFpau) is constitutively synthesized and stored by specific granule-containing hemocyte subpopulations. Fish Shellfish Immunol. 97, 294–299 (2020).
78. Hancock, R. E. W. & Diamond, G. The role of cationic antimicrobial peptides in innate host defences. Trends Microbiol. 8, 402–410 (2000).
79. Destoumieux, D. et al. Penaeidins, a new family of antimicrobial peptides isolated from the shrimp Penaeus vannamei (Decapoda). J. Biol. Chem. 272, 28398–28406 (1997).
80. Amparyup, P., Kondo, H., Hirono, I., Aoki, T. & Tassanakajon, A. Molecular cloning, genomic organization and recombinant expression of a crustin-like antimicrobial peptide from black tiger shrimp Penaeus monodon. Mol. Immunol. 45, 1085–1093 (2008).
81. Destoumieux, D. et al. Penaeidins, antimicrobial peptides with chitin-binding activity, are produced and stored in shrimp granulocytes and released after microbial challenge. J. Cell Sci. 113, 461–469 (2000).
82. Muñoz, M., Vandenbulcke, F., Gueguen, Y. & Bachère, E. Expression of penaeidin antimicrobial peptides in early larval stages of the shrimp Penaeus vannamei. Dev. Comp. Immunol. 27, 283–289 (2003).
83. Bartlett, T. C. et al. Crustins, homologues of an 11.5-kDa antibacterial peptide, from two species of penaeid shrimp, LitoPenaeus vannamei and Litopenaeus setiferus. Mar. Biotechnol. 4, 278–293 (2002).
84. Supungul, P. et al. Antimicrobial peptides discovered in the black tiger shrimp Penaeus monodon using the EST approach. Dis. Aquat. Organ. 61, 123–135 (2004).
85. Antony, S. P. et al. Molecular characterization of a crustin-like antimicrobial peptide in the giant tiger shrimp, Penaeus monodon, and its expression profile in response to various immunostimulants and challenge with WSSV. Immunobiology 216, 184–194 (2011).
86. Smith, V. J. & Dyrynda, E. A. Antimicrobial proteins: From old proteins, new tricks. Mol. Immunol. 68, 383–398 (2015).
87. Gross, P. S., Bartlett, T. C., Browdy, C. L., Chapman, R. W. & Warr, G. W. Immune gene discovery by expressed sequence tag analysis of hemocytes and hepatopancreas in the Pacific White Shrimp, LitoPenaeus vannamei, and the Atlantic White Shrimp, L. setiferus. Dev. Comp. Immunol. 25, 565–577 (2001).
88. Zhang, J., Li, F., Wang, Z. & Xiang, J. Cloning and recombinant expression of a crustin-like gene from Chinese shrimp, Fenneropenaeus chinensis. J. Biotechnol. 127, 605–614 (2007).
89. Somboonwiwat, K. et al. Recombinant expression and anti-microbial activity of anti- lipopolysaccharide factor (ALF) from the black tiger shrimp Penaeus monodon. Dev. Comp. Immunol. 29, 841–851 (2005).
90. Carriel-Gomes, M. C. et al. In vitro antiviral activity of antimicrobial peptides against herpes simplex virus 1, adenovirus, and rotavirus. Mem. Inst. Oswaldo Cruz 102, 469–472 (2007).
91. Yedery, R. D. & Reddy, K. V. R. Identification, cloning, characterization and recombinant expression of an anti-lipopolysaccharide factor from the hemocytes of Indian mud crab, Scylla serrata. Fish Shellfish Immunol. 27, 275–284 (2009).
92. Abnave, P., Muracciole, X. & Ghigo, E. Macrophages in invertebrates: From insects and crustaceans to marine bivalves. Results Probl. Cell Differ. 62, 147–158 (2017).
93. Cerenius, L. & Söderhäll, K. Coagulation in invertebrates. J. Innate Immun. 3, 3–8 (2011).
94. Tsing, A., Arcier, J. M. & Brehélin, M. Hemocytes of Penaeid and Palaemonid shrimps: Morphology, cytochemistry, and hemograms. J. Invertebr. Pathol. 53, 64–77 (1989).
95. Smith, V. J. & Söderhäll, K. Induction of degranulation and lysis of haemocytes in the freshwater crayfish, Astacus astacus by components of the prophenoloxidase activating system in vitro. Cell Tissue Res. 233, 295–303 (1983).
96. Johansson, M. W. & Söderhäll, K. Exocytosis of the prophenoloxidase activating system from crayfish haemocytes. J. Comp. Physiol. B 156, 175–181 (1985).
97. Sarathi, M. et al. Comparative study on immune response of Fenneropenaeus indicus to Vibrio alginolyticus and white spot syndrome virus. Aquaculture 271, 8–20 (2007).
98. Itami, T. et al. Enhancement of disease resistance of kuruma shrimp, Penaeus japonicus, after oral administration of peptidoglycan derived from Bifidobacterium thermophilum. Aquaculture 164, 277–288 (1998).
99. Campa-Córdova, A. I., Hernández-Saavedra, N. Y., De Philippis, R. & Ascencio, F. Generation of superoxide anion and SOD activity in haemocytes and muscle of American white shrimp (LitoPenaeus vannamei) as a response to β-glucan and sulphated polysaccharide. Fish Shellfish Immunol. 12, 353–366 (2002).
100. Gutiérrez-Dagnino, A. et al. Efecto de la inulina y del ácido fúlvico en la supervivencia, crecimiento, sistema inmune y prevalencia de WSSV en LitoPenaeus vannamei. Lat. Am. J. Aquat. Res. 43, 912–921 (2015).
101. Bayne, C. J. Non-Self Phagocytosis Phagocytosis appears to be an ancient line of defense in Invertebrate Recognition. Bioscience 40, 723–731 (1990).
102. Reiber, C. L. & McGaw, I. J. A review of the open and closed circulatory systems: New terminology for complex invertebrate circulatory systems in light of current findings. Int. J. Zool. 2009, (2009).
103. Wang, R., Lee, S. Y., Cerenius, L. & Söderhäll, K. Properties of the prophenoloxidase activating enzyme of the freshwater crayfish, Pacifastacus leniusculus. Eur. J. Biochem. 268, 895–902 (2001).
104. Mai, H. N., Aranguren Caro, L. F., Cruz-Flores, R. & Dhar, A. K. Development of a Recombinase Polymerase Amplification (RPA) assay for acute hepatopancreatic necrosis disease (AHPND) detection in Pacific white shrimp (Penaeus vannamei). Mol. Cell. Probes 57, 101710 (2021).
105. Aguilar-Rendón, K. G., Lozano-Olvera, R., Yáñez-Rivera, B. & Soto-Rodriguez, S. A. Bacteriological and histopathological analysis of Penaeus vannamei experimentally infected with Vibrio parahaemolyticus-AHPND strains. Dis. Aquat. Organ. 140, 167–177 (2020).
106. Jiravanichpaisal, P., Lee, B. L. & Söderhäll, K. Cell-mediated immunity in arthropods: Hematopoiesis, coagulation, melanization and opsonization. Immunobiology 211, 213–236 (2006).
107. Holmblad, T. & Söderhäll, K. Cell adhesion molecules and antioxidative enzymes in a crustacean, possible role in immunity. Aquaculture 172, 111–123 (1999).
108. Owens, L., De Beer, S. & Smith, J. Lymphoidal parvovirus-like particles in Australian penaeid prawns. Dis. Aquat. Organ. 11, 129–134 (1991).
109. Duangsuwan, P. et al. Histological and three dimensional organizations of lymphoid tubules in normal lymphoid organ of Penaeus monodon. Fish Shellfish Immunol. 24, 426–435 (2008).
110. Thomas, A. B. D. L. Handbook of normal penaeid shrimp histology. Aquaculture vol. 76 (1989).
111. Rusaini & Owens, L. Insight into the lymphoid organ of penaeid prawns: A review. Fish Shellfish Immunol. 29, 367–377 (2010).
112. Anggraeni, M. S. & Owens, L. The haemocytic origin of lymphoid organ spheroid cells in the penaeid prawn Penaeus monodon. Dis. Aquat. Organ. 40, 85–92 (2000).
113. Shao, M. Y., Zhang, Z. F., Kang, K. H., Chen, Z. T. & Kim, J. M. The study on the cytology and histochemistry of lymphoid organ spheroids in Penaeus chinensis. Aquaculture 240, 463–471 (2004).
114. Hasson, K. W., Lightner, D. V., Mohney, L. L., Redman, R. M. & White, B. M. Role of lymphoid organ spheroids in chronic Taura syndrome virus (TSV) infections in Penaeus vannamei. Dis. Aquat. Organ. 38, 93–105 (1999).
115. Andrade, T. P. D., Redman, R. M. & Lightner, D. V. Evaluation of the preservation of shrimp samples with Davidson’s AFA fixative for infectious myonecrosis virus (IMNV) in situ hybridization. Aquaculture 278, 179–183 (2008).
116. Rodríguez, J., Ruiz, J., Maldonado, M. & Echeverría, F. Immunodetection of hemocytes, peneidins and α2-macroglobulin in the lymphoid organ of white spot syndrome virus infected shrimp. Microbiol. Immunol. 56, 562–571 (2012).
117. Phyllis T, J. a Review of Fixed Phagocytic and pinocytotic cell of Decapod crustaceans, with remarks on hemocytes. Fish. 11, 679–704 (1987).
118. Horiuchi, S. Characterization of antennary gland Na, K-ATPase in the Freswater Crayfish, Procambarus Clarki Girard. Comp. Biochem. Physiol. 65, 391–394 (1980).
119. Tsai, J. R. & Lin, H. C. Functional anatomy and ion regulatory mechanisms of the antennal gland in a semi-terrestrial crab, Ocypode stimpsoni. Biol. Open 3, 409–417 (2014).
120. Amatul-Samahah, M. A. et al. Vaccination trials against vibriosis in shrimp: A review. Aquac. Reports 18, 100471 (2020).
121. Verschuere, L., Rombaut, G., Sorgeloos, P. & Verstraete, W. Probiotic Bacteria as Biological Control Agents in Aquaculture. Microbiol. Mol. Biol. Rev. 64, 655–671 (2000).
122. Zokaeifar, H. et al. Effects of Bacillus subtilis on the growth performance, digestive enzymes, immune gene expression and disease resistance of white shrimp, LitoPenaeus vannamei. Fish Shellfish Immunol. 33, 683–689 (2012).
123. Romano, N., Koh, C. B. & Ng, W. K. Dietary microencapsulated organic acids blend enhances growth, phosphorus utilization, immune response, hepatopancreatic integrity and resistance against Vibrio harveyi in white shrimp, LitoPenaeus vannamei. Aquaculture 435, 228–236 (2015).
124. Cabello, F. C. Heavy use of prophylactic antibiotics in aquaculture: A growing problem for human and animal health and for the environment. Environ. Microbiol. 8, 1137–1144 (2006).
125. Bray, W. A., Williams, R. R., Lightner, D. V. & Lawrence, A. L. Growth, survival and histological responses of the marine shrimp, LitoPenaeus vannamei, to three dosage levels of oxytetracycline. Aquaculture 258, 97–108 (2006).
126. Flegel, T. W. A future vision for disease control in shrimp aquaculture. J. World Aquac. Soc. 50, 249–266 (2019).
127. Hentzer, M. et al. attenuation of PA by QSI. EMBO J. 22, 3803–3815 (2003).
128. Defoirdt, T., Sorgeloos, P. & Bossier, P. Alternatives to antibiotics for the control of bacterial disease in aquaculture. Curr. Opin. Microbiol. 14, 251–258 (2011).
129. Nealson, K. H., Platt, T. & Hastings, J. W. Cellular control of the synthesis and activity of the bacterial luminescent system. J. Bacteriol. 104, 313–322 (1970).
130. Khmel, I. A., Belik, A. S., Zaitseva, Y. V & Danilova, N. N. Quorum Sensing and Communication in Bacteria. Moscow Univ. Biol. Sci. Bull. 63, 25–31 (2008).
131. Lobo, C. et al. Dietary probiotic supplementation (Shewanella putrefaciens Pdp11) modulates gut microbiota and promotes growth and condition in Senegalese sole larviculture. Fish Physiol. Biochem. 40, 295–309 (2014).
132. Kumar, V., Roy, S., Meena, D. K. & Sarkar, U. K. Application of Probiotics in Shrimp Aquaculture: Importance, Mechanisms of Action, and Methods of Administration. Rev. Fish. Sci. Aquac. 24, 342–368 (2016).
133. Fernandes, S. et al. Immuno-stimulatory effect and toxicology studies of salt pan bacteria as probiotics to combat shrimp diseases in aquaculture. Fish Shellfish Immunol. 113, 69–78 (2021).
134. FULLER, R. Probiotics in man and animals. J. Appl. Bacteriol. 66, 365–378 (1989).
135. Hill, C. et al. Expert consensus document: The international scientific association for probiotics and prebiotics consensus statement on the scope and appropriate use of the term probiotic. Nat. Rev. Gastroenterol. Hepatol. 11, 506–514 (2014).
136. Akhter, N., Wu, B., Memon, A. M. & Mohsin, M. Probiotics and prebiotics associated with aquaculture: A review. Fish Shellfish Immunol. 45, 733–741 (2015).
137. Ringø, E. & Gatesoupe, F. J. Lactic acid bacteria in fish: A review. Aquaculture 160, 177–203 (1998).
138. Gram, L. et al. AH2, a Possible Probiotic Treatment of Fish. Society 65, 969–973 (1999).
139. Toledo, A., Castillo, N. M., Carrillo, O. & Arenal, A. Probióticos: una realidad en el cultivo de camarones. Artículo de revisión. 30, (2018).
140. Moriarty, D. J. W. Control of luminous Vibrio species in penaeid aquaculture ponds. Aquaculture 164, 351–358 (1998).
141. Skjermo, J. & Vadstein, O. Techniques for microbial control in the intensive rearing of marine larvae. Aquaculture 177, 333–343 (1999).
142. Kesarcodi-Watson, A., Kaspar, H., Lategan, M. J. & Gibson, L. Probiotics in aquaculture: The need, principles and mechanisms of action and screening processes. Aquaculture 274, 1–14 (2008).
143. Farzanfar, A. The use of probiotics in shrimp aquaculture. FEMS Immunol. Med. Microbiol. 48, 149–158 (2006).
144. Kongnum, K. & Hongpattarakere, T. Effect of Lactobacillus plantarum isolated from digestive tract of wild shrimp on growth and survival of white shrimp (LitoPenaeus vannamei) challenged with Vibrio harveyi. Fish Shellfish Immunol. 32, 170–177 (2012).
145. Babu, B. et al. Surveillance of disease incidence in shrimp farms located in the east coastal region of India and in vitro antibacterial efficacy of probiotics against Vibrio parahaemolyticus. J. Invertebr. Pathol. 179, 107536 (2021).
146. Newaj-Fyzul, A., Al-Harbi, A. H. & Austin, B. Review: Developments in the use of probiotics for disease control in aquaculture. Aquaculture 431, 1–11 (2014).
147. Knipe, H., Temperton, B., Lange, A., Bass, D. & Tyler, C. R. Probiotics and competitive exclusion of pathogens in shrimp aquaculture. Rev. Aquac. 13, 324–352 (2021).
148. Chomwong, S., Charoensapsri, W., Amparyup, P. & Tassanakajon, A. Two host gut-derived lactic acid bacteria activate the proPO system and increase resistance to an AHPND-causing strain of Vibrio parahaemolyticus in the shrimp LitoPenaeus vannamei. Dev. Comp. Immunol. 89, 54–65 (2018).
149. Šušković, J. et al. Antimicrobial activity - The most important property of probiotic and starter lactic acid bacteria. Food Technol. Biotechnol. 48, 296–307 (2010).
150. Ben Braïek, O. et al. Safety, potential biotechnological and probiotic properties of bacteriocinogenic Enterococcus lactis strains isolated from raw shrimps. Microb. Pathog. 117, 109–117 (2018).
151. Chiu, S. T. et al. Probiotic, Lactobacillus pentosus BD6 boost the growth and health status of white shrimp, LitoPenaeus vannamei via oral administration. Fish Shellfish Immunol. 117, 124–135 (2021).
152. Chen, Y. H. & He, J. G. Effects of environmental stress on shrimp innate immunity and white spot syndrome virus infection. Fish Shellfish Immunol. 84, 744–755 (2019).
153. Kewcharoen, W. & Srisapoome, P. Probiotic effects of Bacillus spp. from Pacific white shrimp (LitoPenaeus vannamei) on water quality and shrimp growth, immune responses, and resistance to Vibrio parahaemolyticus (AHPND strains). Fish Shellfish Immunol. 94, 175–189 (2019).
154. Shen, W. Y., Fu, L. L., Li, W. F. & Zhu, Y. R. Effect of dietary supplementation with Bacillus subtilis on the growth, performance, immune response and antioxidant activities of the shrimp (LitoPenaeus vannamei). Aquac. Res. 41, 1691–1698 (2010).
155. Mirbakhsh, M. et al. Effects of Bacillus subtilis on the water quality, stress tolerance, digestive enzymes, growth performance, immune gene expression, and disease resistance of white shrimp (LitoPenaeus vannamei) during the early hatchery period. Aquac. Int. (2021) doi:10.1007/s10499-021-00758-7.
156. Leonel Ochoa-Solano, J. & Olmos-Soto, J. The functional property of Bacillus for shrimp feeds. Food Microbiol. 23, 519–525 (2006).
157. Abdollahi-Arpanahi, D. et al. Efficacy of two commercial and indigenous probiotics, Bacillus subtilis and Bacillus licheniformis on growth performance, immuno-physiology and resistance response of juvenile white shrimp (LitoPenaeus vannamei). Aquaculture 496, 43–49 (2018).
158. Yilmaz, M., Soran, H. & Beyatli, Y. Antimicrobial activities of some Bacillus spp. strains isolated from the soil. Microbiol. Res. 161, 127–131 (2006).
159. Abriouel, H., Franz, C. M. A. P., Omar, N. Ben & Galvez, A. Diversity and applications of Bacillus bacteriocins. FEMS Microbiol. Rev. 35, 201–232 (2011).
160. García-Medel, D. I. et al. Bacillus licheniformis BCR 4-3 increases immune response and survival of LitoPenaeus vannamei challenged with Vibrio parahaemolyticus IPNGS16. Aquac. Int. 28, 2303–2318 (2020).
161. Phung, L. T., Phuong, T. T. M., Phung, N. K. & Nicolas, M. Isolation of Bacillus strains inhibition disease resistance of Acute Hepatopancreatic Necrosis Disease on the Shrimp in Can Gio, Vietnam. IOP Conf. Ser. Mater. Sci. Eng. 736, (2020).
162. AUSTIN, B., STUCKEY, L. F., ROBERTSON, P. A. W., EFFENDI, I. & GRIFFITH, D. R. W. A probiotic strain of Vibrio alginolyticus effective in reducing diseases caused by Aeromonas salmonicida, Vibrio anguillarum and Vibrio ordalii. J. Fish Dis. 18, 93–96 (1995).
163. Domínguez-Borbor, C. et al. The marine symbiont Pseudovibrio denitrificans, is effective to control pathogenic Vibrio spp. in shrimp aquaculture. Aquaculture 508, 127–136 (2019).
164. Gullian, M., Thompson, F. & Rodriguez, J. Selection of probiotic bacteria and study of their immunostimulatory effect in Penaeus vannamei. Aquaculture 233, 1–14 (2004).
165. Rengpipat, S., Phianphak, W., Piyatiratitivorakul, S. & Menasveta, P. Effects of a probiotic bacterium on black tiger shrimp Penaeus monodon survival and growth. Aquaculture 167, 301–313 (1998).
166. Balcázar, J. L. & Rojas-Luna, T. Inhibitory activity of probiotic Bacillus subtilis UTM 126 against Vibrio species confers protection against vibriosis in juvenile shrimp (LitoPenaeus vannamei). Curr. Microbiol. 55, 409–412 (2007).
167. Chiu, C. H., Guu, Y. K., Liu, C. H., Pan, T. M. & Cheng, W. Immune responses and gene expression in white shrimp, LitoPenaeus vannamei, induced by Lactobacillus plantarum. Fish Shellfish Immunol. 23, 364–377 (2007).
168. Balcázar, J. L., Rojas-Luna, T. & Cunningham, D. P. Effect of the addition of four potential probiotic strains on the survival of pacific white shrimp (LitoPenaeus vannamei) following immersion challenge with Vibrio parahaemolyticus. J. Invertebr. Pathol. 96, 147–150 (2007).
169. Tseng, D. Y. et al. Enhancement of immunity and disease resistance in the white shrimp, LitoPenaeus vannamei, by the probiotic, Bacillus subtilis E20. Fish Shellfish Immunol. 26, 339–344 (2009).
170. Wang, Y. & Gu, Q. Effect of probiotics on white shrimp (Penaeus vannamei) growth performance and immune response. Mar. Biol. Res. 6, 327–332 (2010).
171. NavinChandran, M. et al. Influence of probiotic bacterium Bacillus cereus isolated from the gut of wild shrimp Penaeus monodon in turn as a potent growth promoter and immune enhancer in P. monodon. Fish Shellfish Immunol. 36, 38–45 (2014).
172. Liu, H. et al. Isolation of a putative probiotic strain S12 and its effect on growth performance, non-specific immunity and disease-resistance of white shrimp, LitoPenaeus vannamei. Fish Shellfish Immunol. 41, 300–307 (2014).
173. Sánchez-Ortiz, A. C. et al. Effect of mixed Bacillus spp isolated from pustulose ark Anadara tuberculosa on growth, survival, viral prevalence and immune-related gene expression in shrimp LitoPenaeus vannamei. Fish Shellfish Immunol. 59, 95–102 (2016).
174. Wang, H. et al. Pseudoalteromonas probiotics as potential biocontrol agents improve the survival of Penaeus vannamei challenged with acute hepatopancreatic necrosis disease (AHPND)-causing Vibrio parahaemolyticus. Aquaculture 494, 30–36 (2018).
175. Yi, C. C., Liu, C. H., Chuang, K. P., Chang, Y. T. & Hu, S. Y. A potential probiotic Chromobacterium aquaticum with bacteriocin-like activity enhances the expression of indicator genes associated with nutrient metabolism, growth performance and innate immunity against pathogen infections in zebrafish (Danio rerio). Fish Shellfish Immunol. 93, 124–134 (2019).
176. Wang, Y. et al. Host associated mixed probiotic bacteria induced digestive enzymes in the gut of tiger shrimp Penaeus monodon. Saudi J. Biol. Sci. 27, 2479–2484 (2020).
177. Ock Kim, Y. et al. Growth promoting activity of Penaeus indicus by secondary metabolite producing probiotic bacterium Bacillus subtilis isolated from the shrimp gut. J. King Saud Univ. - Sci. 32, 1641–1646 (2020).
178. Defoirdt, T. Virulence mechanisms of bacterial aquaculture pathogens and antivirulence therapy for aquaculture. Rev. Aquac. 6, 100–114 (2014).
179. Kotzamanidis, C., Kourelis, A., Litopoulou-Tzanetaki, E., Tzanetakis, N. & Yiangou, M. Evaluation of adhesion capacity, cell surface traits and immunomodulatory activity of presumptive probiotic Lactobacillus strains. Int. J. Food Microbiol. 140, 154–163 (2010).
180. Lamari, F., Sadok, K., Bakhrouf, A. & Gatesoupe, F. J. Selection of lactic acid bacteria as candidate probiotics and in vivo test on Artemia nauplii. Aquac. Int. 22, 699–709 (2014).
181. Senok, A. C., Ismaeel, A. Y. & Botta, G. A. Probiotics: Facts and myths. Clin. Microbiol. Infect. 11, 958–966 (2005).
182. Basson, A., Flemming, L. A. & Chenia, H. Y. Evaluation of adherence, hydrophobicity, aggregation, and biofilm development of Flavobacterium johnsoniae-like isolates. Microb. Ecol. 55, 1–14 (2008).
183. Conrad, J. C. Physics of bacterial near-surface motility using flagella and type IV pili: Implications for biofilm formation. Res. Microbiol. 163, 619–629 (2012).
184. Schniederberend, M. et al. Modulation of flagellar rotation in surfaceattached bacteria: A pathway for rapid surface-sensing after flagellar attachment. PLoS Pathog. 15, 1–30 (2019).
185. Pham, D. et al. Selection and characterization of potential probiotic bacteria for Litopenaeus stylirostris shrimp hatcheries in New Caledonia. Aquaculture 432, 475–482 (2014).
186. Ming, L., Zhang, Q., Yang, L. & Huang, J. A. Comparison of antibacterial effects between antimicrobial peptide and bacteriocins isolated from Lactobacillus plantarum on three common pathogenic bacteria. Int. J. Clin. Exp. Med. 8, 5806–5811 (2015).
187. Fakruddin, M., Hossain, M. N. & Ahmed, M. M. Antimicrobial and antioxidant activities of Saccharomyces cerevisiae IFST062013, a potential probiotic. BMC Complement. Altern. Med. 17, 1–11 (2017).
188. Iyapparaj, P. et al. Optimization of bacteriocin production by Lactobacillus sp. MSU3IR against shrimp bacterial pathogens. Aquat. Biosyst. 9, 1–10 (2013).
189. Wang, X. W. & Wang, J. X. Pattern recognition receptors acting in innate immune system of shrimp against pathogen infections. Fish Shellfish Immunol. 34, 981–989 (2013).
190. Ochoa-álvarez, N. A., Casillas-Hernández, R., Magallón-Barajas, F. J., Ramirez-Orozco, J. M. & Carbajal-Millan, E. Protector effect of beta-glucans from shrimp pond-related yeasts in Penaeus vannamei rearing under white spot syndrome virus presence. Lat. Am. J. Aquat. Res. 49, 18–28 (2021).
191. Luan, L. Q., Vu, N. T., Nghia, N. T. & Thao, N. H. P. Synergic degradation of yeast β-glucan with a potential of immunostimulant and growth promotor for tiger shrimp. Aquac. Reports 21, 100858 (2021).
192. Sivakamavalli, J. & Vaseeharan, B. Purification, characterization and functional analysis of a novel β-1, 3-glucan binding protein from green tiger shrimp Penaeus semisulcatus. Fish Shellfish Immunol. 35, 689–696 (2013).
193. Wilson, W. et al. Immune gene expression profile of Penaeus monodon in response to marine yeast glucan application and white spot syndrome virus challenge. Fish Shellfish Immunol. 43, 346–356 (2015).
194. Xu, B. et al. The influence of dietary β-1,3-glucan on growth performance, feed utilization, antioxidative and immune status of Pacific white shrimp, LitoPenaeus vannamei. Aquac. Nutr. 1–12 (2021) doi:10.1111/anu.13299.
195. Vidya Sagar Reddy, G. & Ch, V. Efficacy of β-glucan from Debaryomyces hansenii as an immunostimulant in LitoPenaeus vannamei culture. Aquac. Int. 29, 1451–1458 (2021).
196. Lee, C. L., Chang, C. C., Kuo, H. W. & Cheng, W. Pectin of cacao pod husk, an efficient immunostimulant for white shrimp, LitoPenaeus vannamei. Fish Shellfish Immunol. 107, 357–366 (2020).
197. Li, W. Dscam in arthropod immune priming: What is known and what remains unknown. Dev. Comp. Immunol. 125, 104231 (2021).
198. Thomas, A., Sudheer, N. S., Kiron, V., Bright Singh, I. S. & Narayanan, R. B. Expression profile of key immune-related genes in Penaeus monodon juveniles after oral administration of recombinant envelope protein VP28 of white spot syndrome virus. Microb. Pathog. 96, 72–79 (2016).
199. Gibson, G. R. & Roberfroid, M. B. Dietary modulation of the human colonic microbiota: Introducing the concept of prebiotics. J. Nutr. 125, 1401–1412 (1995).
200. Bindels, L. B., Delzenne, N. M., Cani, P. D. & Walter, J. Opinion: Towards a more comprehensive concept for prebiotics. Nat. Rev. Gastroenterol. Hepatol. 12, 303–310 (2015).
201. Roberfroid, M. B. Nutritional and Health Benefits of Inulin and Oligofructose Concepts in Functional Foods: The Case of Inulin and Oligofructose. J. Nutr 129, 1398–1401 (1999).
202. Guerreiro, I., Oliva-Teles, A. & Enes, P. Prebiotics as functional ingredients: focus on Mediterranean fish aquaculture. Rev. Aquac. 10, 800–832 (2018).
203. Bailey, J. S., Blankenship, L. C. & Cox, N. A. Effect of fructooligosaccharide on Salmonella colonization of the chicken intestine. Poult. Sci. 70, 2433–2438 (1991).
204. Zhou, L. et al. Dietary prebiotic inulin benefits on growth performance, antioxidant capacity, immune response and intestinal microbiota in Pacific white shrimp (LitoPenaeus vannamei) at low salinity. Aquaculture 518, 734847 (2020).
205. Zhou, Z., Ding, Z. & Huiyuan, L. V. Effects of dietary short-chain fructooligosaccharides on intestinal microflora, survival, and growth performance of juvenile white shrimp, LitoPenaeus vannamei. J. World Aquac. Soc. 38, 296–301 (2007).
206. Li, P. et al. Dietary supplementation of short-chain fructooligosaccharides influences gastrointestinal microbiota composition and immunity characteristics of pacific white shrimp, LitoPenaeus vannamei, cultured in a recirculating system. J. Nutr. 137, 2763–2768 (2007).
207. Widanarni, W., Taufik, A., Yuhana, M. & Ekasari, J. Dietary mannan oligosaccharides positively affect the growth, digestive enzyme activity, immunity and resistance against Vibrio harveyi of pacific white shrimp (LitoPenaeus vannamei) larvae. Turkish J. Fish. Aquat. Sci. 19, 271–278 (2019).
208. Ding, Y., Pan, L., Gao, G. & Huang, H. In vitro and in vivo immunologic potentiation of herb extracts on shrimp (LitoPenaeus vannamei). Fish Shellfish Immunol. 107, 556–566 (2020).
209. Lee, P. T. et al. Polysaccharides from the green alga caulerpa racemosa (agardh, 1873) improve the immune response and antioxidant status in the white shrimp LitoPenaeus vannamei (boone, 1931) (dendrobranchiata, penaeidae). Crustaceana 93, 611–632 (2020).
Received: 23 October 2021 / Accepted: 15 February 2022 / Published:15 May 2022
Citation: Ramírez M, Debut A, Alexis F, Rodríguez J. Control of vibriosis in shrimp through the management of the microbiota and the immune system. Revis Bionatura 2022;7(2) 1. http://dx.doi.org/10.21931/RB/2022.07.02.1