2023.08.04.37
Files > Volume 8 > Vol 8 no 4 2023

High microbiological contamination in surface waters in the upper basin of the Choluteca River in Honduras
Victoria Maldonado1,
Keylin Mendoza1, Luis Rivera1, Tania Peña2,
Marcio Chirinos-Escobar2, Lourdes Enríquez1, Gustavo Fontecha1
and Bryan Ortiz1
1.
Instituto de
Investigaciones en Microbiología, Universidad Nacional Autónoma de Honduras
(UNAH)
2.
Tegucigalpa
11101, Honduras.
3.
Instituto
Hondureño de Ciencias de la Tierra, Universidad Nacional Autónoma de Honduras
(UNAH),Tegucigalpa 11101, Honduras.
Corresponding author:
[email protected] Tel: +50433035342
Available
from. http://dx.doi.org/10.21931/RB/2023.08.04.37
ABSTRACT
Microorganisms with a high
pathogenic potential for humans and animals can spread through water. This
represents a severe threat to public health, particularly in low- and
middle-income countries where sanitation conditions are often limited. Due to
their constant presence in the intestine and feces of both people and animals,
total coliforms, thermotolerant coliforms, and intestinal Enterococcus
have historically been employed as indicators of fecal contamination to
evaluate water quality for human use and consumption. Under no circumstances
should water sources for human use and consumption have bacteria indicating
fecal contamination. The objective of this study was to evaluate the
microbiological quality of surface water from six sub-basins located in the
upper basin of the Cholulteca River in Honduras and determine the Escherichia coli phylogroups isolated in these
samples. Our results show high rates of fecal contamination, suggesting that
surface waters in the upper Choluteca River basin are unsafe for human
consumption. Phylogroups B1 and D were the most frequent among 29 E. coli
isolates, while phylogroups C and F were the least frequent. Measures must be taken to raise awareness about sanitation
and good practices for managing household waste and those generated by
agro-industrial and livestock activities in the area. Surface water in the
Choluteca River basin must be adequately treated before consumption because it
may impact people's health. In addition, we encourage prompt decision-making by
the health authorities. In conclusion, this study assessed the
microbiological quality of water in the upper Choluteca River basin. Our
findings show high rates of fecal contamination and the predominance of E. coli
strains from phylogroups associated with fecal contamination, suggesting that
these waters are unsafe for human consumption.
Keywords: fecal contamination; E. coli; phylogroups; surface water; Choluteca River basin; Honduras
Keywords: fecal contamination; E. coli; phylogroups; surface water; Choluteca River basin; Honduras
INTRODUCTION
Surface water is any body of water on the
earth's surface. Surface waters can be lotic when they move in one direction,
such as rivers, springs, and streams, or stagnant lentic waters, such as
wetlands, lakes, ponds, and reservoirs 1, 2. Water is distributed
quite unevenly on the surface of the world. Only 2.5% of surface water is
freshwater, with most of the surface water (about 97%) concentrated in the
seas.
Humans depend critically on surface waters.
Surface water is essential for many daily activities, including livestock farming,
agricultural irrigation, hydroelectricity generation, recreational activities,
and industrial processes. In the same way, surface waters are usually an essential
source of drinking water for human communities and domestic animals. They are a
fundamental pillar for maintaining and developing ecological systems 3.
In recent times, the global use of water has
grown steadily. In 2018, the world demand for water was estimated at 4,600 km3
per year, and it is expected that with the increase in the world population,
this will have an increase of up to 6,000 km3/year, representing an
increase of up to 30% 4. This rise raises concerns about whether enough
water will be available for a significant portion of the global population. Estimates
indicate that by 2050, nearly 3.2 billion people will face acute water scarcity
4, 5. In addition to the increase in the water demand, a continuous
deterioration in its quality has been reported. An example is that about 80% of
the total industrial and municipal wastewater is currently released into the
environment without any prior treatment, and in developing countries, it can
reach 95% 4. As a result of these practices, chemical and biological
contaminants infiltrate water bodies, resulting in a detrimental impact on
ecosystems 4, 6, 7.
As a result of biological pollution, a variety
of potentially hazardous microorganisms, such as bacteria, viruses, helminths,
and protozoa, which are frequently present in the feces of humans and animals,
may spread via surface water. Through leaching, septic tank leaks, sewage, and
industrial waste, these pathogens can access surface water sources
and reach community water supplies 8-10. In most cases, the
transmission of these microorganisms occurs through the fecal-oral route, primarily
through ingesting contaminated water. Less frequent transmission routes include
inhalation or aspiration of water microdroplets and direct exposure by contact,
skin, and mucous membranes during recreational activities 11.
According to the World Health Organization
(WHO), every year, more than 3.4 million people die as a result of
water-related diseases 12. The Global Burden of Disease (GBD) data
estimated that in 2015, an unsafe water source resulted in 1.2 million deaths
and 71.7 million disability-adjusted life years (DALYs), including 1.1 million
deaths and 61.1 million DALYs from diarrheal diseases 13. In the
same way, 34% of the 1.4 million diarrhea-related deaths that took place in
low- and middle-income countries in 2016 were caused by unclean water. Also,
31% were linked to inadequate sewage systems, and 12% were related to
unsanitary behavior. Therefore, access to safe water sources plays an important
role in morbidity and mortality from infectious diseases 14, and fecal
contamination of water bodies intended for human use and consumption
constitutes one of the main health risks 9. Therefore, it is crucial
to regularly assess the quality of surface water and decide how safe it is to
use and consume 15, 16.
Traditionally, bacteriological markers have
been used to monitor and verify the quality of water intended for human use and
consumption, including the presence and count of total coliforms (TC),
thermotolerant coliforms (TtC) and intestinal enterococci (IE) 15-18.
Among the thermotolerant coliforms, Escherichia coli has been considered
the most robust fecal indicator 15, 16, 19-21. Furthermore, the
phylogenetic distribution of E. coli has been suggested as a
complementary analysis to help better understand its presence in waters 17.
E. coli can be classified phylogenetically into 8 groups (A, B1, B2, C,
D, E, F y G). Phylogroups A and B1 are associated with commensal and
antibiotic-resistant strains, while phylogroups B2 and D have been related to
human pathogenic strains 23, 24. B1 predominates in the intestinal
microbiota of animals, while B2 has been identified as the main phylogroup in
human feces. In contrast, E. coli isolates considered
"naturalized," intestinal isolates that have adapted to the wild over
time are classified primarily into cryptic clades 17. To the best of our knowledge, there is scarce information
about the surface water quality in Honduras. This study aimed to determine the
phylogenetic distribution of the E. coli isolates present in these waters and
evaluate the microbiological quality of surface water from six sub-basins in
the upper Choluteca River basin in the central part of Honduras.
MATERIALS AND METHODS
Sampling
sites
The Choluteca River originates in
central Honduras, flows through Francisco Morazán, El Paraíso, and Choluteca
departments, and drains into the Gulf of Fonseca in the Pacific Ocean. The
study area is located in the central-southern region of the country, with a dry
forest transition climate. It is delimited by the upper part of the Choluteca
River basin (Figure 1), encompassing the capital city, Tegucigalpa.
Water samples from surface sources
were collected between October 2019 and April 2022 at 29 randomly chosen
locations in six sub-basins spanning ten municipalities (Figure 1). The
number of samples collected in each sub-basin was distributed as follows:
Choluteca alta (n = 8), Yeguare (n = 7), Guacerique (n = 6), Río Chiquito (n =
3), Río del Hombre (n = 3), San José (n = 2) (Figure 1). The water samples were
collected following the indications of the Standard Methods for the Examination
of Water and Wastewater, 23rd Edition 18. 500 mL of water
was collected from each sampling location in sterile plastic bags, which were
then transported in refrigerators at 4 °C until analysis.
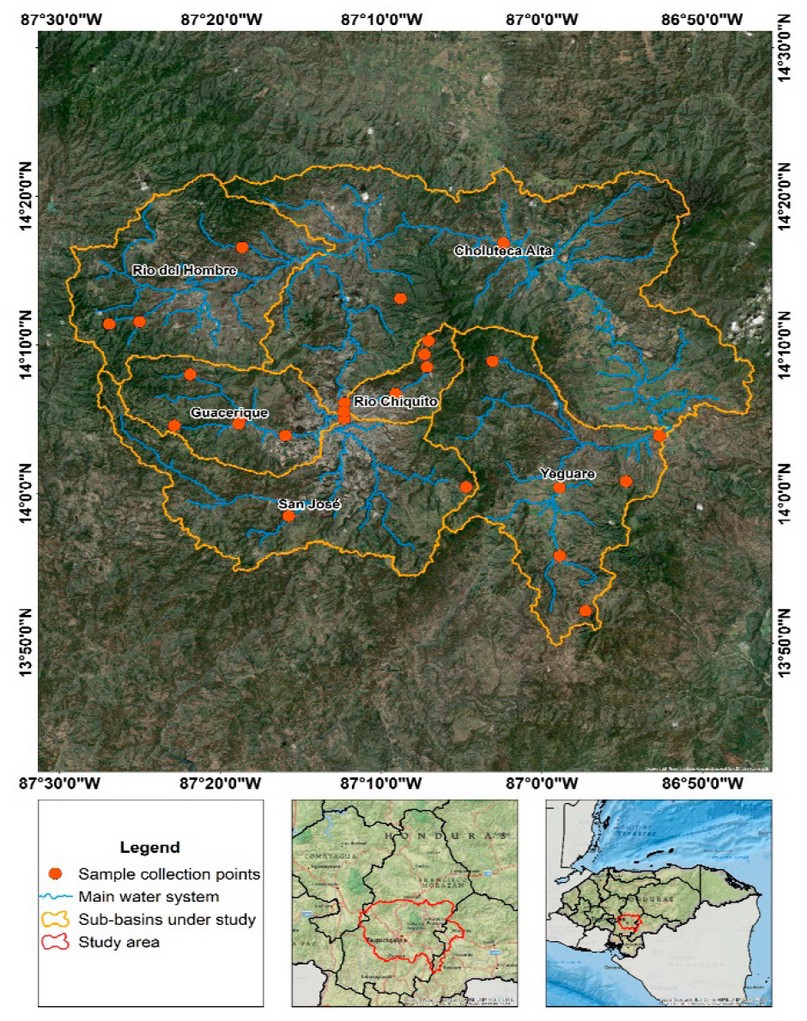
Figure 1. Map showing the geographic location
of the sub-basins under study and sampling sites. Scale 1:150,000, geographic
coordinate system, WGS 84 ellipsoid.
Determination of Fecal Contamination Indicators
The water samples were processed
within the first 6 hours after collection. Following the instructions of the
Standard Methods for the Examination of Water and Wastewater, 23rd
Edition 18, the membrane filtration technique was used to isolate
and count total coliforms (TC), thermotolerant coliforms (TtC), and intestinal Enterococcus
(IE) present in the samples. 500 mL of water was collected from each sampling
location in sterile conditions and passed through filters with cellulose
nitrate membranes with pore sizes of 0.45 µm and a diameter of 47 mm (Millipore
Inc®).
Once the samples were filtered, the
membranes were transferred to Petri dishes with m-Endo LES agar (Criterion™)
for the search for TC. Moreover, mFC agar (Criterion™) was used to search for
TtC and m-Enterococcus agar (Acumedia®) to search for intestinal
enterococci. The incubation conditions were 24 h at 37 °C for TC, and 24 h at
44.5 °C for TtC. The m-Enterococcus medium was incubated at 37 °C for 48 h.
Once the incubation period had elapsed, the number of Colony Forming Units
(CFU)/100 mL was determined.
Dark red colonies with a metallic
luster were interpreted as TCs in the m-Endo medium. Blue colonies in the mFC
medium were considered TtC; in the case of IE, any red colony was interpreted
as Enterococcus spp.
E. coli phenotypic identification
Identification of bacterial species
was carried out using previously reported protocols 22. Briefly, any
characteristic colony presumptively identified as E. coli was selected
randomly from each sample, cultured on MacConkey agar and blood agar media, and
incubated at 37 °C for 18-24 h. A presumptive identification was made based on
traditional biochemical tests Indole, Mobility, Voges Proskauer, and Simmons
Citrate. All the biotypes that presented any of the patterns, (+ + – –) or (– +
– –), were confirmed using the API 20 E identification system (BioMerieux,
Marcy-l’Étoile, France). Bacteria identified as E. coli were inoculated
into Brain Hearth Infusion (BHI) broth with 20% glycerol and stored at –80 °C
for further studies.
DNA Extraction and Identification of Phylogenetic Groups
The strains were inoculated in
Luria-Bertani liquid medium for DNA extraction and incubated for 24 h at 37 ºC.
Subsequently, the genomic DNA was extracted using the extraction kit Wizard
Genomic DNA Purification (Promega, Madison, Wisconsin, USA.), according to the
manufacturer's instructions. Finally, the DNA was eluted in 100 μL of
the buffer TE. The extracted DNA was stored at -20 °C until use.
The methodology previously described
by Clermont et al. 2013 and Clermont et al. 2019 was used 23, 24 for
identifying phylogenetic groups. This approach is based on detecting the genes arpA,
chuA, yjaA, TspE4.C2, trpA, ybgD, and cfaB. PCR amplification
reactions were performed according to previously published protocols 22.
RESULTS AND DISCUSSION
In this study, 29 surface water sources located
throughout the upper Choluteca River basin were tested for the presence of
total coliforms (TC), thermotolerant coliforms (TtC), and intestinal Enterococcus
(IE). Table 1 shows all TC, TtC, and IE counts by sub-basin and municipality.
The presence of TC was observed in 100% of the
water samples. TC counts ranged from 1.3 × 101 CFU/100 mL to 4.2 ×
107 CFU/100 mL. The highest TC count was observed in three samples from
three subbasins (Guacerique, San José, and Choluteca Alta), collected during
the rainy season of 2021. TC counts in these samples were higher than 1 × 107
CFU/100 mL. On the other hand, two samples from the Río Chiquito and
Yeguare sub-basins, which are in the municipalities of the Central District and
Tatumbla, respectively, showed the lowest counts (1.3 × 101 and 7.6
× 101 CFU/100 mL).
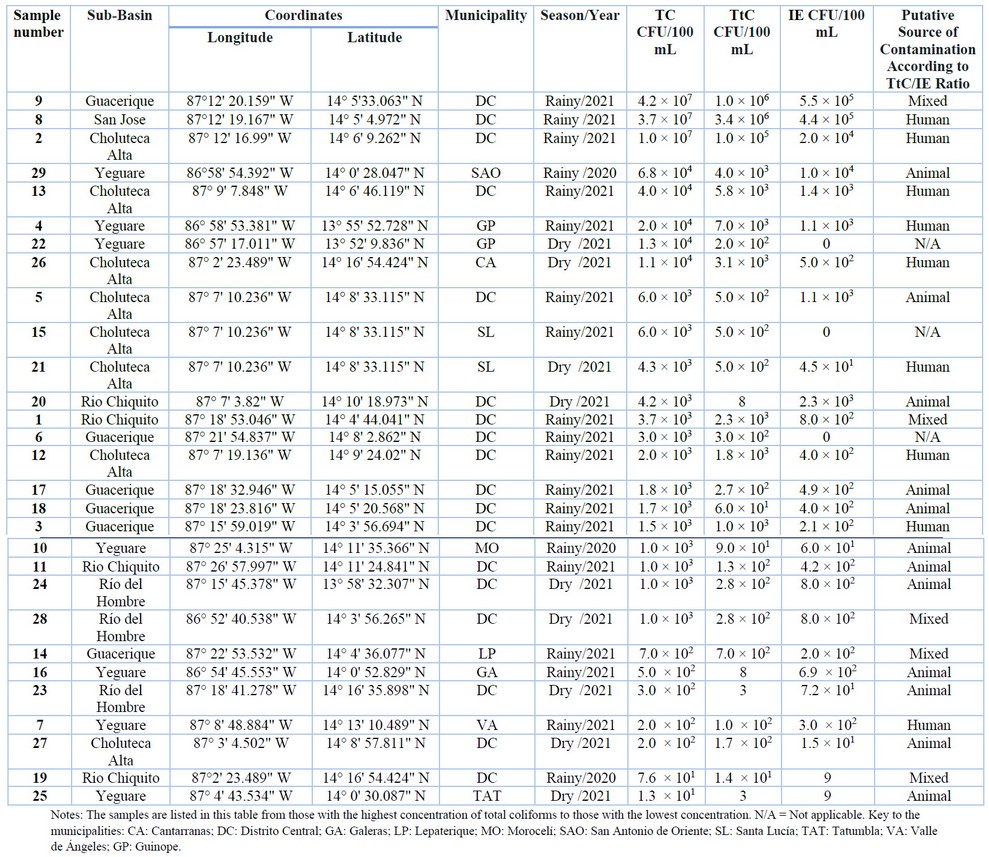
Table 1. Counts
of Total Coliforms (TC), Thermotolerant Coliforms (TtC), and Intestinal Enterococcus
(IE) and the result of the analysis of the TtC/IE ratio in the Choluteca River
basin of Honduras
TC detection is a tool to assess the overall
sanitary quality of water supplies 25. Detection of TC in surface
waters suggests contamination from sewage discharges or decomposing matter,
especially organic waste. These are usually associated with the lack of septic
systems, sewage leaks, sewage systems in poor condition, or inadequate waste
management from agriculture and livestock around sampling points 15, 21,
26, 27.
On the other hand, the presence of TtC was
observed in all samples. Their count varied between 3 CFU/100 mL to 3.4 × 106
CFU/100 mL. The highest count of TtC was observed in the San José and
Guacerique sub-basins, located in the municipality of the Central District. The
counts at these points were equal to or greater than 1 × 107. In
contrast, four samples from the Yeguare (n = 2), Río Chiquito (n = 1), and Río
del Hombre (n = 1) sub-basins showed the lowest TtC counts, with values of less
than 1 × 101 CFU/100 mL. The distribution of TC, TtC, and IE depends
on sub-basin, is shown in Figure 2.
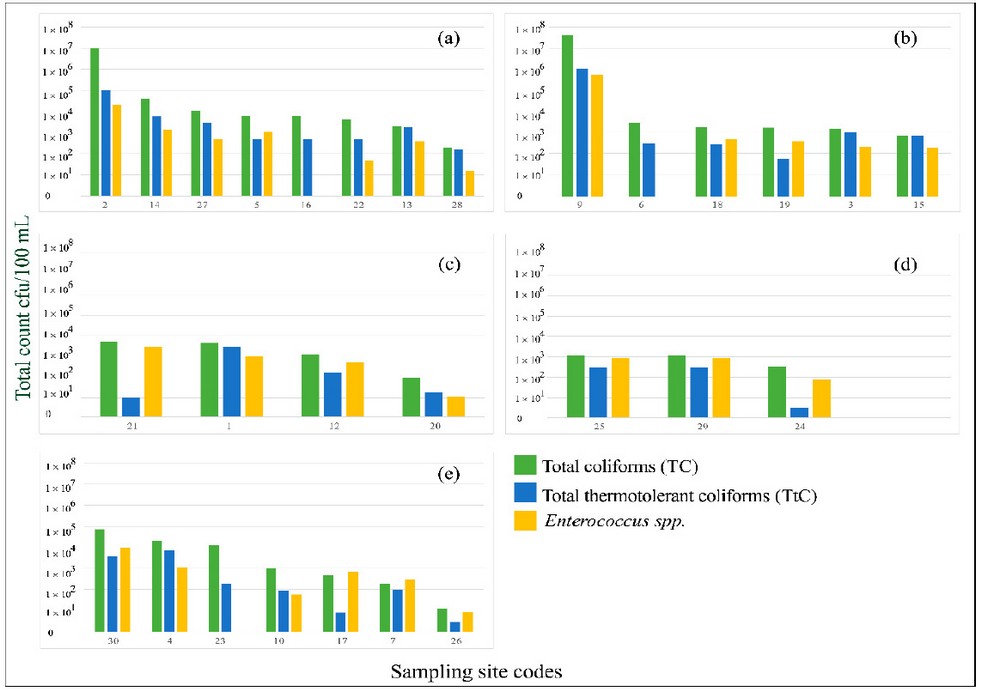
Figure 2. Counts of total coliforms (green bars),
thermotolerant coliforms (blue bars), and intestinal Enterococcus (yellow bars)
by sampling point distributed by sub-basin: (a) Choluteca alta, (b) Guacerique,
(c) Río Chiquito, (d) Río del Hombre, (e) Yeguare.
It has been established that E. coli
accounts for around 95% of TtC isolates in waters 25, 28, 29. For
this reason, E. coli is an accurate indicator of fecal contamination due
to its constant presence in the intestine and feces of warm-blooded animals.
The presence of TtC in any body of water has a high predictive value for the
potential presence of other pathogenic microorganisms for humans 19-21, 30.
On
the other hand, it has been suggested that the presence of IE might be used as
a supplementary test for assessing water quality. In this investigation, 90% of
the sampling locations revealed the presence of IE. IE counts ranged from 9
CFU/100 mL to 5 × 105 CFU/100 mL. On the other hand, the IE count
was 0 in three sampling locations from the Choluteca Alta, Guacerique, and
Yeguare sub-basins.
According to various hypotheses, human
excretion of TtC, IE, and E. coli differs significantly from that of
animals in quantity 31-34. According to what was previously proposed
by Geldreich et al. 32, we assessed the source of microbiological
contamination through the relationship between thermotolerant coliforms and
intestinal enterococci (TtC / IE) in this study. In brief, a TtC/IE ratio
greater than 4.0 indicates contamination with a human origin, while a ratio
less than 0.7 indicates contamination with an animal origin. On the other hand,
mixed contamination is considered to exist when the TtC/IE ratio ranges from
0.7 to 4.0 32. Following this criterion, the origin of the
contamination was determined for those sites whose IE counts were more
significant than or equal to 1 CFU/100 mL. 90% (n=26) of the 29 samples
examined had levels higher than or equal to 1 CFU/100 mL. The analysis of the
microbiological contamination source is shown in Table 1. The most frequent
source of contamination was determined to be of mixed origin in 19.2% of the
samples. In comparison, the TtC/IE ratio suggested human contamination in 34.6%
of the cases and animal origin in 46.1% of the 26 samples studied.
The potential health risks associated with
using and consuming water from the various sampling locations were also
explored in this study. The WHO has established four categories of potential
health risks related to E. coli or thermotolerant coliform (TtC)
concentrations. These risk categories are low (<1 CFU/100 mL), intermediate
(1–10 CFU/100 mL), high (11–100 CFU/100 mL), and very high (>100 CFU/100 mL)
15. Our findings indicate
that 100% of the samples did not meet the WHO drinking water quality recommendations
and that 58.6% constituted a high risk for human consumption. Likewise, 10.3%
were categorized as high risk, while 13.7% were intermediate risk. No sampling
point was suitable for consumption following the WHO risk guidelines.
The phylogenetic distribution of the E. coli
strains isolated from the various sample points was also examined. The
predominant phylogenetic group was A (31%), followed by D (24.2%), clade I
(17.2%), B1 (13.8%), B2 (10.3%) and phylogroup E with 3.4%. Intestinal E.
coli isolates that are "naturalized," or to have adapted to
natural habitats, are primarily grouped in cryptic clades 17. Therefore,
the low prevalence of these cryptic clades and many phylogroups associated with
animals and humans suggests a high anthropogenic activity close to the sampling
points.
Here, we have evidence of high microbiological
contamination in the surface waters of the upper Choluteca River basin, with a
high anthropogenic influence. Human and animal waste, improper wastewater
management practices, and runoff from nearby metropolitan areas and the agricultural
sector are all potential sources of water contamination. Microorganisms that
suggest fecal contamination should never be present in water intended for human
consumption 15, 18, 25. The presence of microorganisms with the
potential to cause disease in drinking water usually results in significant adverse
effects on public health. The most common waterborne organisms, such as
cholera, bacillary dysentery, typhoid fever, gastroenteritis, leptospirosis,
amoebic dysentery, cryptosporidiosis, giardiasis, and balantidiasis, could be
transmitted through water surface of the upper Choluteca River basin.
On the other hand, surface waters are not
isolated components of the hydrological system. The hydrologic interactions
between surface and subsurface waters occur by subsurface lateral flow through
the soil and infiltration into or exfiltration from the saturated zones. In
this regard, a high load of microbial contaminants in surface waters may impact
groundwater 35, 36. The 2030 sustainable development agenda's goal
of achieving universal and equitable access to drinking water is significantly
hampered by such contamination 37 since groundwater is the primary
source of drinking water for half of the world's population 38, 39.
This potential pathway for cross-contamination between surface water and
groundwater could explain previously published results of high rates of fecal
contamination found in groundwater in the upper Choluteca River basin 22.
These data should not go unnoticed since about
1.5 million people, representing more than 14% of the country's total
population, benefit directly or indirectly from the surface and groundwater of
the upper Choluteca River basin. In summary, due to the high rates of fecal
pollution found in our study, which most likely result from biological waste
from people and animals, immediate local and state-level action is required to
monitor and control the water quality.
CONCLUSIONS
The microbiological quality of the water in the
upper basin of the Choluteca River was evaluated in this study. Our results
indicate that these waters are inappropriate for human consumption due to the
high rates of fecal contamination and the prevalence of E. coli strains
from phylogroups linked to fecal contamination. Actions must be taken to raise
public awareness of sanitation-related challenges and effective procedures for
handling waste from households, livestock, and the agricultural sector.
Additionally, as the water in the Honduran Choluteca River basin can
potentially impact people's health, it must be safely treated before
consumption. Likewise, we suggest prompt intervention by decision-makers
Author
Contributions: LE, KM, GF and TP conceptualized the
study; VM, KM, BO, M.C.-E. and LR obtained the isolates and performed the
experiments; BO, KM, LR and GF organized and cured the data; writing and
original draft preparation, BO and GF; all the authors contributed with
writing, review, and editing the manuscript; supervision, project
administration, and funding acquisition, KM, TP and LE All authors have read
and agreed to the published version of the manuscript.
Funding: This research was funded by Fondo Global
para el Medio Ambiente (Global Environmental Facility: GEF)/Programa de las
Naciones Unidas (PNUD), Project "Adaptación basada en Ecosistemas en el
Corredor Boscoso Central", grant number 00094142. APC was covered by DICIHT, UNAH.
Institutional Review Board Statement: Not applicable
Acknowledgments: Not applicable
Conflicts of
Interest: The authors declare no conflict of
interest.
REFERENCES
1. Winter TC. Groundwater and surface
water: a single resource: Diane Publishing; 2000.
2. Hayashi M, Rosenberry DO. Effects of
Ground Water Exchange on the Hydrology and Ecology of Surface Water.
Groundwater. 2002;40(3):309-16.
3. Centers for Disease Control and
Prevention Waterborne Disease. The Many Uses of Water 2021 [Available
from: https://www.cdc.gov/healthywater/other/index.html, (accessed on 13
February 2023).
4. WWAP (United Nations World Water
Assessment Programme)The United Nations World Water Development Report 2018
Nature-Based Solutions for Water. Paris,. Unesco, Paris; 2017.
5. Burek P, Satoh Y, Fischer G, Kahil M,
Scherzer A, Tramberend S, et al. Water futures and solution-fast track
initiative. 2016.
6. Ślósarczyk K, Jakóbczyk-Karpierz S,
Różkowski J, Witkowski AJ. Occurrence of Pharmaceuticals and Personal Care
Products in the Water Environment of Poland: A Review. Water. 2021;13(16):2283.
7. Kurwadkar S. Occurrence and
distribution of organic and inorganic pollutants in groundwater. Water Environ
Res. 2019;91(10):1001-8.
8. Sharma S, Sachdeva P, Virdi JS.
Emerging waterborne pathogens. Applied Microbiology and Biotechnology. 2003;61(5):424-8.
9. Ashbolt NJ. Microbial Contamination of
Drinking Water and Human Health from Community Water Systems. Curr Environ
Health Rep. 2015;2(1):95-106.
10. Mahmud ZH, Islam MS, Imran KM, Hakim SAI,
Worth M, Ahmed A, et al. Occurrence of Escherichia coli and faecal coliforms in
drinking water at source and household point-of-use in Rohingya camps,
Bangladesh. Gut Pathog. 2019;11(1):1-11.
11. World Health Organization. Guidelines for
safe recreational water environments. Volume 2, Swimming pools and similar
environments: World Health Organization; 2006.
12. World Health Organization. Water for
health: taking charge. World Health Organization (WHO); 2001.
13. Forouzanfar MH, Afshin A, Alexander LT,
Anderson HR, Bhutta ZA, Biryukov S, et al. Global, regional, and national
comparative risk assessment of 79 behavioural, environmental and occupational,
and metabolic risks or clusters of risks, 1990–2015: a systematic analysis for
the Global Burden of Disease Study 2015. Lancet. 2016;388(10053):1659-724.
14. Prüss-Ustün A, Wolf J, Bartram J, Clasen
T, Cumming O, Freeman MC, et al. Burden of disease from inadequate water,
sanitation and hygiene for selected adverse health outcomes: an updated
analysis with a focus on low-and middle-income countries. Int J Hyg Environ
Health. 2019;222(5):765-77.
15. Guidelines for drinking-water quality.
Vol. 3, Surveillance and control of community supplies. Vol. 3,
Vigilancia y control de los abastecimientos de agua a la comunidad. 2nd ed.
Geneva: World Health Organization; 1997.
16. Ministerio
de Salud Republica de Honduras. Norma tecnica para la calidad del agua potable
Honduras. Available
online:
http://www.aguasdesiguatepeque.com/imagenes/Archivos%20PDF/Norma%20Tecnica%20calidad%20del%20agua%20potable.pdf.
(accessed on 18 march 2023). 1995.
17. Devane ML, Moriarty E, Weaver L, Cookson
A, Gilpin B. Fecal indicator bacteria from environmental sources; strategies
for identification to improve water quality monitoring. Water Res. 2020;185:116204.
18. Baird R, & Bridgewater, L. . Standard
methods for the examination of water and wastewater. 23rd
edition. Washington, DC: American Public Health Association. 2017.
19. Ramos‐Ramírez
LdC, Romero‐Bañuelos CA, Jiménez‐Ruíz EI, Palomino‐Hermosillo YA,
Saldaña‐Ahuactzi Z, Martínez‐Laguna Y, et al. Coliform bacteria in san Pedro
lake, western Mexico. Water Environ Res. 2021;93(3):384-92.
20. Castro
Fernández MF, Cárdenas Manosalva IR, Colmenares Quintero RF, Montenegro Marín
CE, Diaz Cuesta YE, Escobar Mahecha D, et al. Multitemporal Total Coliforms and
Escherichia coli Analysis in the Middle Bogotá River Basin,
2007–2019. Sustainability. 2022;14(3):1769.
21. Ercumen A, Pickering AJ, Kwong LH, Arnold
BF, Parvez SM, Alam M, et al. Animal feces contribute to domestic fecal
contamination: evidence from E. coli measured in water, hands, food, flies, and
soil in Bangladesh. Environ Sci Technol. 2017;51(15):8725-34.
22. Mendoza
K, Ortiz B, Rivera L, Peña T, Chirinos-Escobar M, Enríquez L, et al. Monitoring of Microbial
Contamination of Groundwater in the Upper Choluteca River Basin, Honduras.
Water. 2023;15(11):2116.
23. Clermont O, Christenson JK, Denamur E,
Gordon DM. The Clermont Escherichia coli phylo-typing method revisited:
improvement of specificity and detection of new phylo-groups. Environ Microbiol
Rep. 2013;5(1):58-65.
24. Clermont O, Dixit OVA, Vangchhia B,
Condamine B, Dion S, Bridier-Nahmias A, et al. Characterization and rapid
identification of phylogroup G in Escherichia coli, a lineage with high virulence and
antibiotic resistance potential. Environ Microbiol. 2019;21(8):3107-17.
25. Water S, Organization WH. WHO guidelines
for drinking water quality: training pack. 2000.
26. Valenzuela M, Lagos B, Claret M, Mondaca
MA, Pérez C, Parra O. Fecal contamination of groundwater in a small rural
dryland watershed in central Chile. Chilean Journal of Agricultural Research. 2009;69(2):235-43.
27. Akpataku KV, Gnazou MD, Nomesi TYA, Nambo
P, Doni K, Bawa LM, et al. Physicochemical and Microbiological Quality of
Shallow Groundwater in Lomé, Togo. Journal of Geoscience and Environment
Protection. 2020;8(12):162.
28. Bartram J, Ballance R. Water quality
monitoring: a practical guide to the design and implementation of freshwater
quality studies and monitoring programmes: CRC Press; 1996.
29. Quality F-PWGoRW, Health C, Canada W.
Guidelines for Canadian Recreational Water Quality: Health and Welfare Canada; 1992.
30. Barrantes
K, Chacón L, Morales E, Rivera-Montero L, Pino M, Jiménez AG, et al. Occurrence of pathogenic
microorganisms in small drinking-water systems in Costa Rica. J Water Health. 2022;20(2):34455.
31. Borrego A, Romero P. Study of the
microbiological pollution of a Malaga littoral area II. Relationschip between
fecal coliforms and fecal streptococci VIèJournée Étude Pollutions, Cannes,
France. 1982:561-9.
32. Geldreich EE, Kenner BA. Concepts of
fecal streptococci in stream pollution. Journal (Water Pollution Control
Federation). 1969:R336-R52.
33. Bisimwa AM, Kisuya B, Kazadi ZM, Muhaya
BB, Kankonda AB. Monitoring faecal contamination and relationship of
physicochemical variables with faecal indicator bacteria numbers in Bukavu
surface waters, tributaries of Lake Kivu in Democratic Republic of Congo.
Hygiene and Environmental Health Advances. 2022;3:100012.
34. Coyne MS, Howell J. The fecal
coliform/fecal streptococci ratio (FC/FS) and water quality in the bluegrass
region of Kentucky. 1994.
35. Sophocleous M. Interactions between
groundwater and surface water: the state of the science. Hydrogeology Journal. 2002;10(1):52-67.
36. Some S, Mondal R, Mitra D, Jain D, Verma
D, Das S. Microbial water pollution with special reference to coliform bacteria
and their nexus with environment. Energy Nexus. 2021;1:100008.
37. Cepal
N. Agenda 2030 y los Objetivos de Desarrollo Sostenible: una oportunidad para
América Latina y el Caribe. 2018.
38. Velis M, Conti KI, Biermann F.
Groundwater and human development: synergies and trade-offs within the context
of the sustainable development goals. Sustain Sci. 2017;12:1007-17.
39. Morris BL, Lawrence AR, Chilton P, Adams
B, Calow RC, Klinck BA. Groundwater and its susceptibility to degradation: a
global assessment of the problem and options for management. 2003.
Received: 26 September 2023 / Accepted:
15 April 2023 / Published:15 December 2023
Citation: Maldonado V,. Mendoza K, Rivera L, Peña T, Chirinos-Escobar M,
Enríquez L, Fontecha G and Ortiz B. High microbiological
contamination in surface waters in the upper basin of the Choluteca River in
Honduras. Revis Bionatura 2023;8
(4) 37. http://dx.doi.org/10.21931/RB/2023.08.04.37
Publisher's Note: Bionatura stays
neutral concerning jurisdictional claims in published maps and institutional
affiliations.
Copyright: © 2023 by
the authors. Submitted for possible open-access publication under the terms and
conditions of the Creative Commons Attribution (CC BY) license
(https://creativecommons.org/licenses/by/4.0/).