2023.08.02.44
Files > Volume 8 > Vol 8 No 2 2023

Plants anatomically engineered by nanomaterials
Osama Abdul-Rahman Owied1 , Muthik Abd Muslim Guda 2,*
, Hawraa Imad Taher 3 , Muslim Abd Ali Abdulhussein 3

1 Faculty of Medicine, University of Sumer, Iraq; [email protected].
2 Department of Ecology, Faculty of Sciences, University of Kufa, Iraq.; [email protected].
3 Department of Horticulture, Faculty of Agriculture, University of Kufa, Najaf, Iraq; [email protected].
* Correspondence: [email protected].
Available from: http://dx.doi.org/10.21931/RB/2023.08.02.44
ABSTRACT
Anatomical characteristics are essential in determining the stress that affects plants. In addition, they provided a piece of evidence for environmental pollution. The increasing use of nanomaterials (EnNos) in industries, medicine, agriculture, and all fields. Nanomaterials also have many uses as a new science; they have toxic effects that have not been studied well. Therefore, this research was interested in recording recent studies on (EnNos) and their impact on the anatomical characteristics of plants.
Moreover, the possibility of using anatomical characteristics as evidence of nano contamination (nanotoxicity) in plants comprises a crucial living component of the ecosystem. Studies on the effect of EnNos (carbon) on plant anatomy indicated that excess EnNos content affects the anatomical structure of the plant from the vital structures of the root, stem and leaves. Toxicological effect on xylem and phylum vessels from toxicological studies to date, Toxicological effects on EnNos of various kinds can be toxic if they are not bound to a substrate or freely circulating in living systems. Different types of EnNos, behavior, and plant capacity generate different paths. Moreover, different, or even conflicting, conclusions have been drawn from most studies on the interactions of EnNos with plants. Therefore, this paper comprehensively reviews studies on different types of carbon EnNos and their interactions with different plant species at the anatomical responses.
Keywords: Anatomical characteristics, nanomaterials, nanotoxicity, Fullerene and Carbon Nanotubes
INTRODUCTION
The Term Of Nanotechnology, Nanotechnology means the generation, processing and diffusion of nanomaterials (Nos), a wide range of applications 1. It became essential for industrial growth in all countries 2. (Nos) are known as nanotechnology-derived products 3. The increase of nanoparticles in recent years and their use in different ways 4. Stimulating by Nanomaterials, the (EnNos) development in agricultural applications led to the development of genetically modified crops (GMCs), livestock production, biopesticides, and tissue culture 6. Because they are low-cost, field application techniques 1-5. Studies have proven that nano-fertilizers stimulate seed germination in addition to enhancing production. Researchers report a significant knowledge gap about the effects of (NOs) on food crops such as vegetables and grains 7. Accumulation and uptake depend on plant type, chemical composition, and size of (EnNos) 8. Some plants are capable of absorbing and accumulating stimuli (Nos). Plant cell interaction with (Nos) alters plant gene expression and associated biological pathways, ultimately influencing plant anatomy 9. The effects of (Nos) on different plant species can vary significantly with different plant shapes, methods and duration of exposure and depend on (Nos) shape, size, chemical composition, concentration, and solubility 10. More studies are needed on the potential risks of using (Nos) and their potential adverse effects 11. Research indicates that (Nos) are highly toxic to aquatic life, bacteria, and human cells at the nanoscale; according to particle physics and studies of micro-atmospheric pollutants, even usually benign substances may become dangerous. Size range of nanomaterials that remain suspended for days to weeks in the air and are respired in plant respiratory system 12; therefore, of particular concern is the ability of nanoparticles to be directly taken up by individual cells and cell nuclei. Bioaccumulation of (Nos) important concern 10-12. Since the physical characteristics of the endoscopic variants are not good, it is difficult to give a definitive opinion on the health and environmental risks 13-16. Therefore, plant cells are determined by the interactions and modified plant gene expression (Nos). They have linked biological pathways, which ultimately influence seedling growth and development. This is due to different effects on seedling growth due to the unique properties of (Nos) that can modify its physical and chemical properties, depending on the surface area and quantum properties 17-20. wherefore, (Nos) must have all properties such as effective concentration with high potency, stability and solubility, and time-controlled release in response to specific stimuli 21-25. The vast majority of the research work done for each product (Nos) and product in agriculture is from it to investigate its stimulating potential before it is considered safe26.
MATERIALS AND METHODS
Stimulating By Ennos On Plant Anatomy
The plants interacting with (Nos) is possible and increased with increasing application and variety of instruments and goods. A plant can accumulate toxic elements inside tissues usually, and intolerant species suffer effects that can lead to death27-30. as well as absorbs essential and nonessential elements, which may induce a certain concentration31. (Nos) can enter the plant body directly or indirectly, or by pollutants in the air, water or soil, which leads to the transfer of (Nos) to the food chains through food. This route is primarily responsible for the transfer of nanotoxicity to the plant-related food chain.32-36 Research studies on selenium have found that selenium-laden plants can supply selenium deficiency in ruminants and other animals, even in very narrow and low toxicity and deficiency states37-39. Other studies have focused on this aspect, including radish (Raphanus sativus), corn (Zea mays), lettuce (Lactuca sativa), cucumber (Cucumis sativus), rape (Brassica napus), and many more19. Most studies have focused on Nos' uptake, accumulation, transport and biotransformation because biodegradation is difficult to trace in living organisms.
RESULTS
Plant Anatomy Response By Carbon Based
Carbon-based (Nos) is total production that led to release in living systems and greater possibilities of adverse environmental effects 41. fullerene C70, fullerol (C60(OH)20), and carbon nanotubes are most studied materials. All of them are highly hydrophobic and able to aggregate; it could accumulate in the living system42. This led to an increased capability to interact with many organic substances. Some plants can uptake mechanisms and accumulation (Nos). Appropriately functionalized (Nos) provided better penetration through the cuticle. This, in turn, allows for a slow and governed discharge of active ingredients on reaching the target weed. For instance, appropriately functionalized lipophilic nano silica gets absorbed into the cuticular lipids of insects by physisorption, damages the protective wax layer, and induces death by desiccation43-45.
Plant Anatomy Response By Fullerene
The individual fullerenes nanoparticle is believed to enter the plant roots through osmotic pressure, capillary forces, and pores in the cell walls by the intercellular plasmodesmata or using the significantly regulated symplastic routes 46. Only the fullerene particles with a diameter of less than the pore diameter of the cell wall could pass through and reach the plasma membrane 47.
Plant Anatomy Response By Fullerol
The small size and hydrophobicity properties inducing a permeability of fuller through the cell wall pores in the plant cell suspension leads to minimal uptake of the (Nos) 48. Consequently, the fuller has accumulated at the interface between the cell wall and plasma membrane 49. This accumulation also occurred between adjacent epidermal cell walls, showing its apoplectic mode of transport in the plant tissues 50-52.
Plant Anatomy Response By Carbon Nanotubes
Carbon nanotubes (CNTs) may possibly have single or multiple carbons layers established in a cylinder 53-55. CNTs behave as fibers, with their properties very different from bulk carbon or graphite 56. Thus, CNTs possess excellent tensile strength and are possibly the strongest, smallest fiber known 57-59. Most studies are increasingly carried out to obtain the uptake and transport mechanism of carbon-based (Nos) into intact plant cells 60. CNTs have phytotoxic effects on plant cells due to aggregation and cause cell death in a dose-dependent manner 61. Cell death is demonstrated by electrolyte leakage and the swelling of the cell plant. Theoretically, a single-wall carbon nanotube is too large to penetrate the cell wall. However, the evidence of an endocytosis-like structure of the plasma membrane in an Arabidopsis thaliana leaf cell indicates the existence of carbon nanotubes. It is exceptionally relevant to guide additional studies with other edible plants 62-64. Then, researches with cell suspensions of Nicotiana tabacum cv. Bright Yellow found that the water-soluble single-wall carbon nanotube with a length of less than 500nm has penetrated the intact cell wall and membrane over fluidic phase endocytosis 65-66. Due to their small size, carbon nanotubes tend to interact with the polysaccharides and proteins in the cell wall and elicit hypersensitive retorts mimicking plant pathogens, leading to cell mortality 80–83.This is supported by recognizing monovalent interactions involving rice mortality and carbon nanotubes. Thus, CNTs could improve root growth of cucumbers (Cucumis sativus), onions (Allium cepa), and nanotube sheets formed by both functionalized-CNTs and no functionalized CNTs on root surfaces, but none entered the roots 67-68.
Plant Anatomy Response By Mwcnts
Multiwall carbon nanotubes (MWCNTs) are 1mm long and 20 nm in diameter 69. MWCNTs are taken up by the seeds and roots system by creating new pores and water uptake to develop tomato seedlings 70. MWCNTs are visualized to be on the root surface before eventually piercing the epidermal and root hair cell walls and cap of the seedlings 31. Furthermore, some studies described that MWCNTs permeate tomato seeds and boost the germination rate by improving the seed water uptake 71-72. Other researchers pointed out that the cell walls of rice cell suspension restrict the entry of the MWCNTs into the cellular cytoplasm, forming black clumps that strongly wrap around and associate with the cells 32. The presence of the clumps, with an increase in the concentration of carbon (Nos), would increase in size and number 31. This hypersensitive response is thought to prevent the entry of MWCNTs through the plant cell walls 32. For example, the seeds treated with MWCNTs showed a few aggregate nanotubes in the vascular system and none in the tissues.
Plant Anatomy Response By Swcnts
The dimension of a typical single-walled carbon nanotube (SWCNT) is about 1 to 2 nm in diameter and 0.1 𝜇m in length 73. Some studies indicate that carbon-based (Nos) surface modifications increased its widespread, dispensability, and water column stability 74-75. Contrarily, no uptake of SWCNTs and its functionalized roots of cucumber seedlings are found after treatment for 84 h. In the form of a nanotube sheet, the SWCNTs were found to adhere to the external surface of the primary and secondary roots 76. However, current results are insufficient to determine the translocation of SWCNTs from the root systems to the aerial parts of the plant 77.
Plant Anatomy Response By Graphene
Graphene is an inert carbon with a two-dimensional crystalline shape with a strong bond of a single carbon atom layer. Studies indicated that at concentrations higher than (1000 mg/L), spinach and red cabbage root hair growth decreased compared to the control plants, although it was inactive 78. This may be due to the accumulation of free radicals, especially H2O2, which increases with increasing concentration and duration of exposure. It also increased necrosis and permeability of organisms, causing significant electrolyte leakage (oxidative stress) 28. For example, the intracellular reduction-oxidation system probably has an essential function in the Induction of cell death induced by graphene 79. It described the accumulation of graphene as leading to cell death, shown by electrolyte leakage from cells 80. Via graphene treatment, the root surface area of cabbage significantly improved, and it may be that an excess of graphene resulted in swelling in Origanum vulgare and Origanum 80-83. Graphene is known to induce phytotoxic effects in plant cells due to its accumulation mechanism. This causes cell death and accumulation in a dose-dependent manner 84.
A similar growth pattern was observed in tomato, cabbage, and red spinach using graphene (Nos) in 38. At higher concentrations of graphene (1000mgL−1), the root hair growth of red spinach and cabbage compared to control plants was reduced 85. Overproduction associated with the accumulation induced by graphene could produce substantial Plant anatomy inhibition, and biomass reduction reported that the production of accumulation could be a primary factor in the toxicological effects of nanostructured materials 40. Declaration of accumulation production by means of H2O2 visualization, visible signs of necrotic damage lesions, and evidence of a massive electrolyte leakage all indicated an oxidative stress mechanism mediated through the necrotic pathway, which requires further study 89-90. The assessment of graphene-stimulated targets terrestrial plant species 86-88. It applies a prolonged exposure period with different concentrations to measure potential risks. I will quote my master's research findings to support the findings of this article.
Effect of ZnO NPs on anatomical trials of studied wild plants
Response of studied wild plants leave cross-section to ZnO NPs treatments
The Scanning electron microscope (SEM) photograph analysis of the studied wild plants' leave cross-section revealed an anatomical structure typical of Dicotyledonous in the control variant (Figure 1). The structure of the leaf tissue was characterized by an ordered organization and the cell's uniform localization in the chlorenchyma of the leaf. The division of mesophyll cells into palisade and spongy parenchyma was remotely traced. 91-93 The parenchyma cells are located in several rows between the upper and lower epidermal layers, characterized by the round form. As mentioned earlier, an insignificant proportion of tightly contacting cells and an extensive intercellular space were established for the cells of parenchyma spatial organization. The vascular bundles comprise about four sectors, the xylem, phloem, parenchyma and bundle sheath (Figure 1- 12 ). However, there were anatomical differences in the dimensions of the bundles in different treatments. These differences were selected for comparison (Figure 1-12).
Response of Peganum harmala leave cross-section
The (SEM) photograph of Peganum harmala plants leave cross-section revealed in the control variant (Figure 1). A uniform localization in the leaf's parenchyma characterized the leaf tissue's structure. The round form characterized the cells of the parenchyma. The vascular bundles comprise about four sectors, the xylem, phloem, parenchyma and bundle sheath (Figure 1 ).
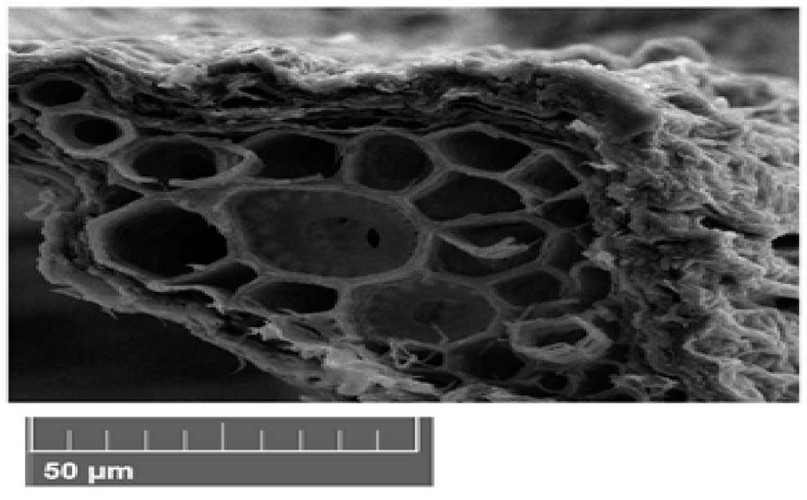
Figure 1. Scanning electron microscope images of Peganum harmala leave cross-section under control treatments. A- epidermis, B- parenchyma, C- phloem, D- xylem.
Treatment of 150 mg/L showed a noticeable increase in the leaf cross section due to the increase in the layers of parenchyma. The vascular bindle increased in number, especially the vessels of xylem and phloem (Figure 2).
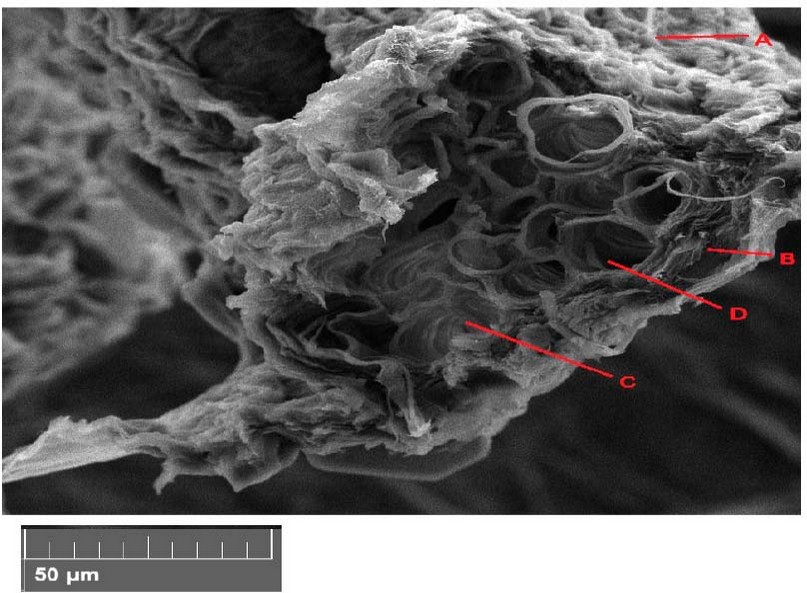
Figure 2. Scanning electron microscope images of Peganum harmala leave cross-section with 150 mg/l treatments. A- epidermis, B- parenchyma, C- phloem, D- xylem.
P eganum harmala leave cross-section with 300 mg/l treatments showed decreased parenchyma cell size. The vascular bindle decreased in number, especially the vessels of xylem (Figure 3).
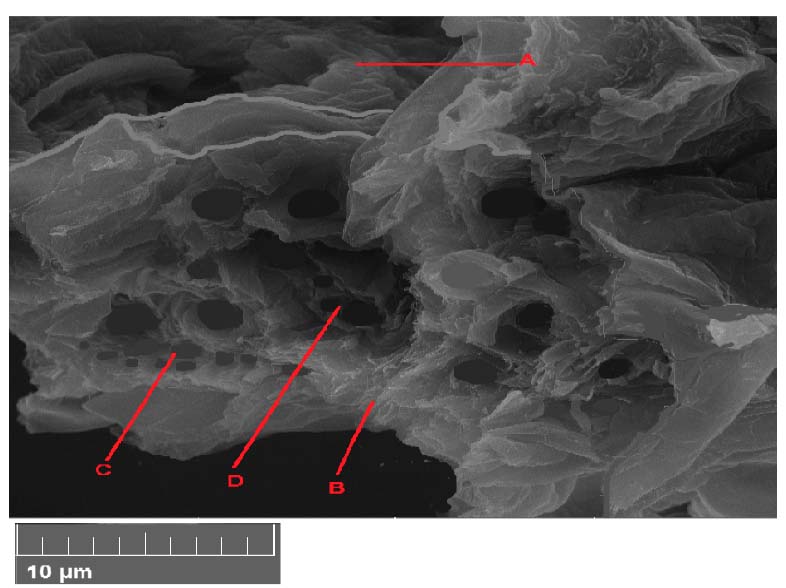
Figure 3. Scanning electron microscope images of Peganum harmala leave cross-section with 300 mg/l treatments. A- epidermis, B- parenchyma, C- phloem, D- xylem.
Figure 4 at the end of the experiment showed decay in the parenchyma tissue, vascular bundle and increased epidermis shrivel of Peganum harmala leave cross-section with 600 mg/l treatments.
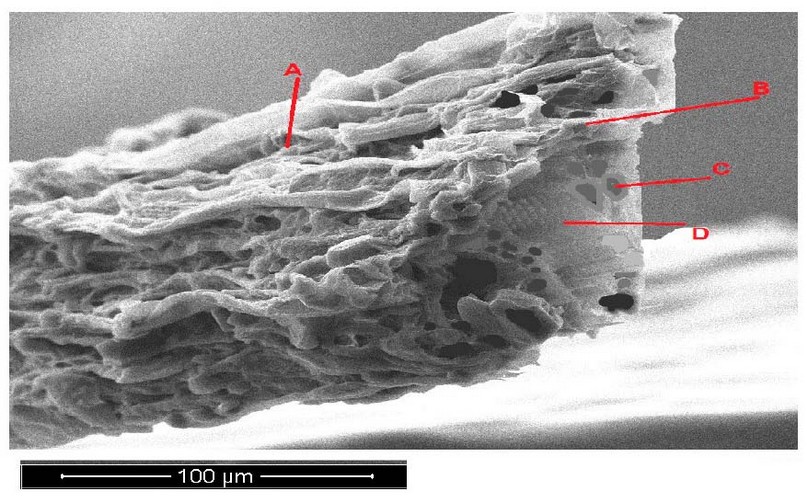
Figure 4. Scanning electron microscope images of Peganum harmala leave cross-section with 600 mg/l treatments. A- epidermis, B- parenchyma, C- phloem, D- xylem.
Response of Portulaca oleracea leave cross-section
The (SEM) photograph of Portulaca oleracea plants leave a cross-section with control treatments (Figure 5). The structure of the parenchyma of the leaf consists of several layers. The cells of the parenchyma were round form. The vascular bundles comprise the xylem, phloem, and parenchyma (Figure 5).
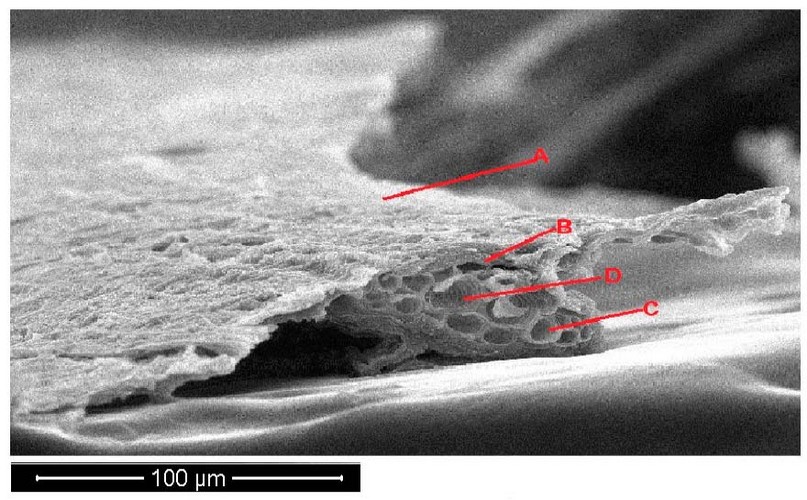
Figure 5. Scanning electron microscope images of Portulaca oleracea leave cross-section under control treatments. A- epidermis, B- parenchyma, C- phloem, D- xylem.
The Portulaca oleracea leave cross-section with 150 mg/l treatments appeared normal leave surface and increased cell wall thickness. The plants appeared smaller in vascular bundle diameter, increased in parenchyma cell size, and decreased in number (Figure 6).
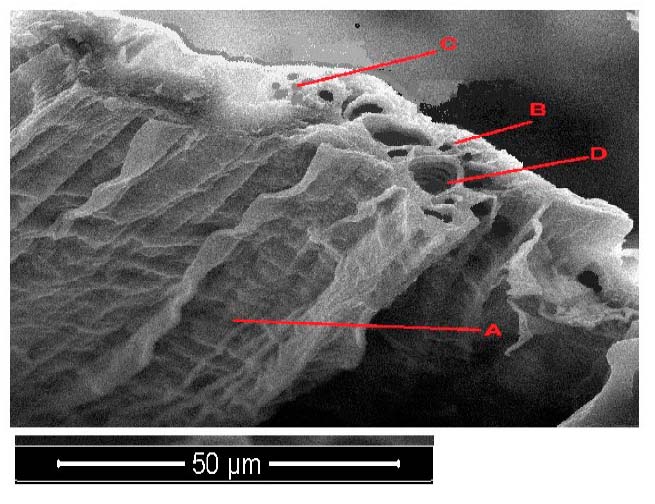
Figure 6. Scanning electron microscope images of Portulaca oleracea leave cross-section with 150 mg/l treatments. A- epidermis, B- parenchyma, C- phloem, D- xylem.
The Portulaca oleracea leave cross-section with 300 mg/l treatments appeared to shrivel in leave surface and increase cell walls thickness. The plants appeared smaller in vascular bundle diameter and increased in epidermis shrivel and started disintegration aerenchyma, which increased in size and decreased in number due to dissolved cell walls (Figure 7).
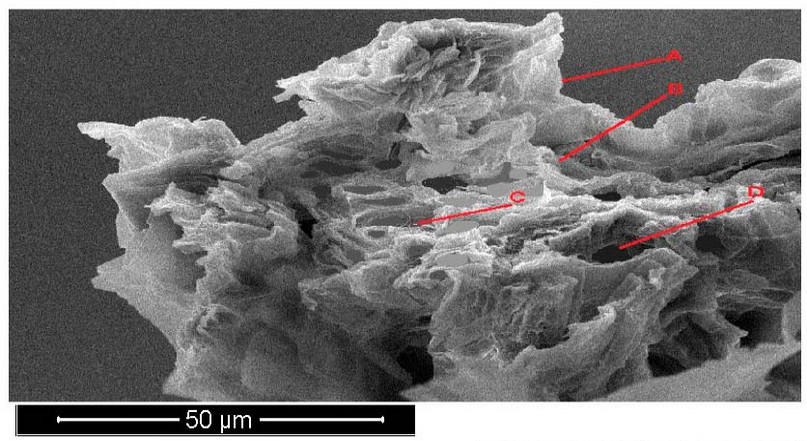
Figure 7. Scanning electron microscope images of Portulaca oleracea leave cross-section with 300 mg/l treatments. A- epidermis, B- parenchyma, C- phloem, D- xylem.
The Portulaca oleracea leave cross-section with 600 mg/l treatments appeared to fade in the leave epidermis cell with folded layer. The plants appeared smaller in vascular bundle diameter and decreased in number due to dissolved cell walls (Figure 8).
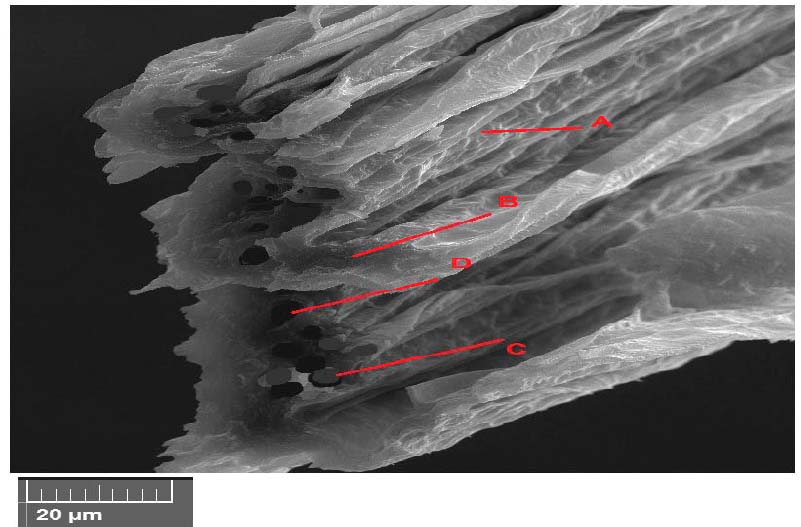
Figure 8. Scanning electron microscope images of Portulaca oleracea leave cross-section with 600 mg/l treatments. A- epidermis, B- parenchyma, C- phloem, D- xylem.
Response of Lepidium sativum leave cross-section
The (SEM) photograph of Lepidium sativum plants leave cross-section revealed in the control variant (Figure 9). A uniform localization in the leaf's parenchyma characterized the leaf tissue's structure. The round form characterized the cells of the parenchyma. The vascular bundles consist of about four sectors, the xylem, phloem, parenchyma and bundle sheath (Figure 9 ).
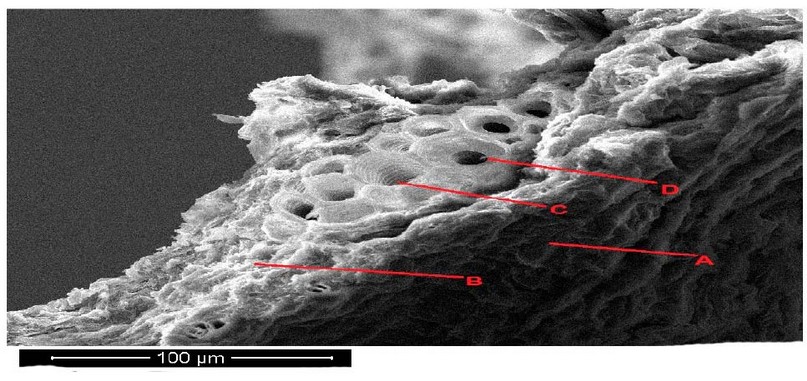
Figure 9. Scanning electron microscope images of Lepidium sativum leave cross section under control treatments. A- epidermis , B- parenchyma, C- phloem, D- xylem.
Lepidium sativum leave cross section with 150 mg/l treatments showed accumulation of ZnO NPs particles on the surface of the plants. Epidermis of Lepidium sativum not arrangement with folded shape. Epidermis cells small and had different shape (Figure 10).
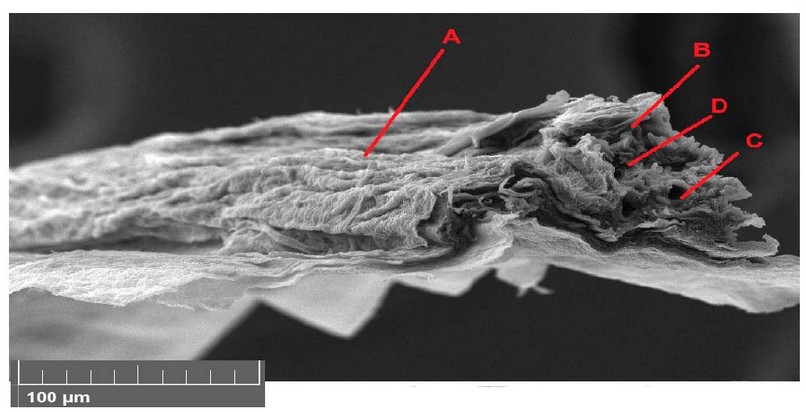
Figure 10. Scanning electron microscope images of Lepidium sativum leave cross-section with 150 mg/l treatments. A- epidermis, B- parenchyma, C- phloem, D- xylem.
The Lepidium sativum leave cross-section with 300 mg/l treatments appeared Multi-layered epidermis and parenchyma with small cell size. The vascular cylinder occupied the central part of the cross-section and appeared to be few and of small diameter in xylem vessels. (Figure 11).
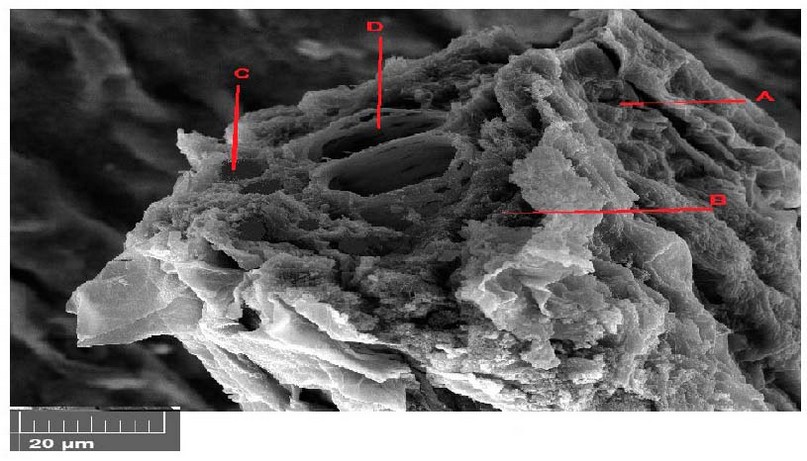
Figure 11. Scanning electron microscope images of Lepidium sativum leave cross-section with 300 mg/l treatments. A- epidermis, B- parenchyma, C- phloem, D- xylem.
SEM images of various macroscopic magnification forces showing plants in 12 Figure. The plant appeared to decay in the parenchyma tissue with shriveling of the epidermis tissue and necrosis in vascular bundle due to the degradation of photosynthesis pigments and deposition of materials in the parenchyma tissue was observed by increasing the pigmentation of the cells and increasing the cell wall thickness (Figure 12).
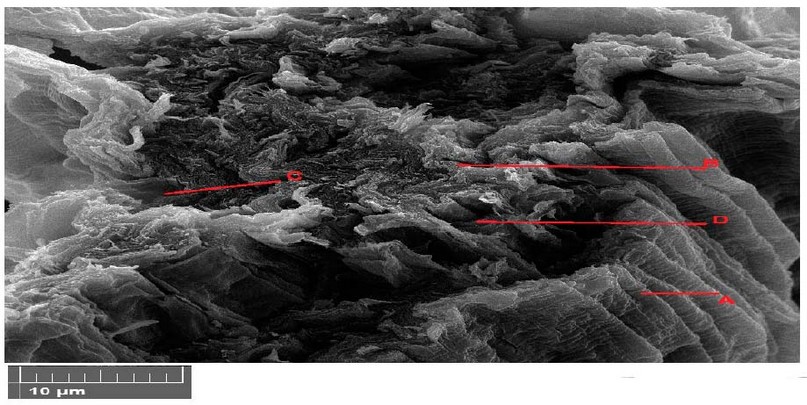
Figure 12. Scanning electron microscope images of Lepidium sativum leaves cross section with 600 mg/l treatments. A- epidermis, B- parenchyma, C- phloem, D- xylem.
DISCUSSION
The only low surface friction of carbon nanotubes is required to assist the flow of organic substances into the cytoplasm94. The Fullerene aggregates were mainly present in or near the stem's vascular system and leaves, whereby the roots have been devoid of fullerene 95. The fullerene aggregation in leaves indicated that they followed the transmission route of nutrients and water through the xylem 96. It has been reported that the presence of fullerene in the form of black aggregates is more plentiful in seeds and roots compared to the leave sand stems for rice seeds 22. There is proof that CNTs could translocate to systemic sites, such as fruits, leaves and roots, which could involve a strong interaction with the cells of the tomato seedling. This resulted in significant changes in whole fruits, leaves, and roots gene expression 79. Though CNTs were discovered to diminish root growth in tomato plants, recent works reported that CNTs penetrated tomato seed coats and significantly enhanced plant anatomy 98.
Meanwhile, in the zucchini species, no adverse effects were noticed root elongation within the examined range of MWCNTs 99. There is proof that graphene could translocate to systemic sites, such as fruits, roots, and leaves, which interact strongly with tomato seedlings' cells, leading to substantial modifications in total gene expression in fruits, leaves, and roots and exerting toxic effects 38. With that, it is unexpected to find the toxic effects of graphene on terrestrial plant species in tomato, cabbage, and red spinach 100
Anatomical indicators showed that the Peganum harmala plant showed the best response compared to the rest of the studied plants, 101 where the stomata remained open, and the xylem and phloem vessels were intact despite being damaged by an increase in the concentration of zinc nanoparticles102. The most studied plant affected was Lepidium sativum, where the stomata were closed and decreased in number, and damage to the vascular system50.
The zinc nano ions entered the cell walls' structure and damaged the cellular organelles. The treatment of 600 mg/L harmed the vascular cylinder (xylem and phloem), causing a decrease in the size of the parenchyma cells. The epidermis is limp, and the number of stomata was decreased and smaller in size, which may lead to their complete closure.103
These results agree with Nemček et al.54 studies that reported a high content of Zn (1200 mg/kg dry weight) was determined in barley leaves grown in pots amended with 30 mmol/kg of Zn in the form of ZnSO4, whereas plants treated with ZnO (NPs) showed higher contents of Zn in the root tissues55,56.57. During the dissolution of ZnO in the root zone, nanosized particles were reported to be absorbed more rapidly than larger-sized particles, such as bulk ZnO particles. Near-edge X-ray absorption spectroscopy showed that ZnO (NPs) of 40 nm were dissolved by roots more quickly than their larger-size counterparts104.
The results demonstrate that most Zn taken up was derived from Zn 2+ released from ZnO (NPs), and Zn accumulated in the form of Zn phosphate. ZnO (NPs) were observed mainly in the epidermis, a small fraction of ZnO (NPs) were present in the cortex cells, and some further entered the vascular system through the sites of the plasmodesmata pore.61,62 However, small amounts of ZnO nanoparticle was observed to translocate to the vascular system, possibly due to the dissolution and transformation of ZnO (NPs) inside the plants105-107.
CONCLUSIONS
The extrapolation of the researcher's opinions into nanotoxicity on plant anatomy led to the conclusion that exceeding the limits required for plant tolerance leads to harmful effects on cell walls, cell division, plant's ability to transport and vital activities, especially Transport units in plants (phloem and xylem) and thus affect negatively on growth. But the information so far is lacking about the permissible limits for accumulating (EnNos). This requires more research for each type of (EnNos) that differ in their physical and chemical properties and multiple uses. Plant species also differ in their response to nanotoxicity. Currently, the use of (EnNos) should be reduced to avoid its adverse effects on plants and the ecosystem.
Funding: "This research received no external funding."
Institutional Review Board Statement: "Not applicable." for studies not involving humans or animals. You might also exclude this statement if the study did not involve humans or animals.
Acknowledgments: This work was supported by the Department of Environmental and Pollution Science, Faculty of Science.
Conflicts of Interest: The funders had no role in the design of the study; in the collection, analyses, or interpretation of data; in the writing of the manuscript, or in the decision to publish the results".
REFERENCES
1 Delgado-Ramos G. C., Nanotechnology in Mexico: global trends and national implications for policy and regulatory issues, Technology in Society, 2014, vol. 37, no. 1, pp. 4–15.
2 Safari J. and Zarnegar Z., Advanced drug delivery systems: nanotechnology of health design A review, Journal of Saudi Chemical Society, 2014, vol. 18, no. 2, pp. 85–99.
3 Vilela Neto O. P., Intelligent computational nanotechnology: the role of computational intelligence in the development of nanoscience and nanotechnology, Journal of Computational and Theoretical Nanoscience, 2014, vol. 11, no. 4, pp. 928–944.
4 M. Guda, , M.Taher, , & B.Almayahi, Anatomical characteristics of vascular bundles associated with heat tolerance in Phragmites australis. Analele Universitatii din Oradea, Fascicula Biologie, 2019, vol.26(2), pp.136-139.
5 M. A.Guda, , A. S.Yoness, , S. J.Mohammed, , & K. K. Alasedi, Enzymatic and anatomical responses of wheat (Triticum aestivum L.) Cultivar IPA 99 to irrigation with magnetized water. Iranian Journal of Ichthyology, 2021. vol.8, pp.280-289.
6 M. A. Guda, , J. K.Obaid, , H. J.Alkurdi, , G. L.Hakeem, , H. M.Salih, , & H. F. A. Isawi, The role of street and park trees to CO2 removal and improving air quality and climate in urban areas in Najaf (Iraq), In IOP Conference Series: Materials Science and Engineering , 2020, Vol. 870, No. 1, p. 012087. IOP Publishing. https://iopscience.iop.org/article/10.1088/1757-899X/870/1/012087/pdf
7 M. A.Guda, , J. K. Obaid, , K.K.Alasedi, , F. J. Alduhaidahawi, Comparison of Vascular plant leaves in the ability to collect Atmospheric heavy metals in urban areas, International Journal of Pharmaceutical Research, 2020. Vol.13(1), p.47-52.
DOI: 10.31838/ijpr/2021.13.01.014
8 V. Dutschk, T. Karapantsios, L. Liggieri, N. McMillan, R. Miller, and V. M. Starov, Smart and green interfaces: from single bubbles/drops to industrial environmental and biomedical applications, Advances in Colloid and Interface Science, 2014,vol. 209, pp. 109–126,.
9 A. M. Al-Halafi, Nanocarriers of nanotechnology in retinal diseases, Saudi Journal of Ophthalmology, 2014.
10 M. Safiuddin, M. Gonzalez, J. W. Cao, and S. L. Tighe, Stateof- the-art report on use of nanomaterials in concrete, International Journal of Pavement Engineering, 2014.
11 X. Zhou, M. Torabi, J. Lu, R. Shen, and K. Zhang, Nanostructured energetic composites: synthesis, ignition/combustion modeling, and applications, ACS Applied Materials and Interfaces, 2014, vol. 6, no. 5, pp. 3058–3074.
12 R. H. Baughman, A. A. Zakhidov, and W. A. de Heer, Carbon nanotubes, the route toward applications, Science, 2002, vol. 297, no. 5582, pp. 787–792.
13 X. Lang, A. Hirata, T. Fujita, and M. Chen, Nanoporous metal/oxide hybrid electrodes for electrochemical supercapacitors, Nature Nanotechnology, 2011,vol. 6, no. 4, pp. 232–236.
14 L. Rizzello and P. P. Pompa, Nanosilver-based antibacterial drugs and devices: mechanisms, methodological drawbacks, and guidelines, Chemical Society Reviews, 2014, vol. 43, no. 5, pp. 1501–1518.
15 K. Yang and Y. Ma, Computer simulation of the translocation of (Nos) with different shapes across a lipid bilayer, Nature Nanotechnology, 2010, vol. 5, no. 8, pp. 579–583,.
16 J. Y. Ljubimova and E. Holler, "Biocompatible nanopolymers: the next generation of breast cancer treatment? Nanomedicine, 2012, vol. 7, no. 10, pp. 1467–1470.
17 D. Astruc, "Electron-transfer processes in dendrimers and their implication in biology, catalysis, sensing and nanotechnology, Nature Chemistry, 2012, vol. 4, no. 4, pp. 255–267.
18 S. Bandyopadhyay, J. R. Peralta-Videa, and J. L. Gardea- Torresdey, Advanced analytical techniques for the measurement of nanomaterials in food and agricultural samples: a review, Environmental Engineering Science, 2013, vol. 30, no. 3, pp.118–125.
19 G. C. Philip, B. Keith, I. Masa, and A. Zettl, Extreme oxygen sensitivity of electronic properties of carbon nanotubes, Science, 2000, vol. 287, no. 5459, pp. 1801–1804.
20 M. K. Mani, G. Viola, M. J. Reece, J. P. Hall, and S. L. Evans, Fabrication of carbon nanotube reinforced iron based magnetic alloy composites by spark plasma sintering, Journal of Alloys and Compounds, 2014, vol. 601, pp. 146–153.
21 A. Hazarika and T. K. Maji, Strain sensing behavior and dynamic mechanical properties of carbon nanotubes/nanoclay reinforced wood polymer nanocomposite, Chemical Engineering Journal, 2014, vol. 247, pp. 33–41.
22 D. Liu, Y. Zhang, X. Sun, and P. R. Chang, Recent advances in bio-sourced polymeric carbohydrate/nanotube composites, Journal of Applied Polymer Science, 2014,vol. 131, no. 12.
23 M.Chai, F. Shi, R. Li,L.Liu,Y.Liu, andF. Liu, Interactive effects of cadmium and carbon nanotubes on the growth and metal accumulation in a halophyte Spartina alterniflora (Poaceae), PLANT ANATOMYRegulation, 2013, vol. 71, no. 2, pp. 171–179.
24 M. V. Khodakovskaya, B. Kim, J. N. Kim et al., Carbon nanotubes as plant anatomy regulators: effects on tomato growth, reproductive system, and soil microbial community, Small, 2013, vol. 9, no. 1, pp. 115–123.
25 Z. Long, J. Ji,K.Yang, D. Lin, and F. Wu, Systematic and quantitative investigation of the mechanism of carbon nanotubes' toxicity toward Algae, Environmental Science and Technology, 2012, vol. 46, no. 15, pp. 8458–8466,.
26 C. Lin, B. Fugetsu, Y. Su, and F. Watari, Studies on toxicity of multi-walled carbon nanotubes on Arabidopsis T87 suspension cells, Journal of Hazardous Materials, 2009, vol. 170, no. 2-3, pp. 578– 583.
27 C.-X. Shen, Q.-F. Zhang, J. Li, F.-C. Bi, and N. Yao, "Induction of programmed cell death in Arabidopsis and rice by single-wall carbon nanotubes, American Journal of Botany, 2010, vol. 97, no. 10, pp. 1602–1609.
28 M. V. Khodakovskaya, K. De Silva, A. S. Biris, E. Dervishi, and H.Villagarcia, Carbon nanotubes induce growth enhancement of tobacco cells, ACS Nano, 2012, vol. 6, no. 3, pp. 2128–2135.
29 H. Yi, S. Nisar, S. Lee et al., Patterned assembly of genetically modified viral nanotemFigures via nucleic acid hybridization, Nano Letters, 2005, vol. 5, no. 10, pp. 1931–1936.
30 E. Ca˜nas, M. Long, S. Nations et al., Effects of functionalized and nonfunctionalized single-walled carbon nanotubes on root elongation of select crop species, Environmental Toxicology and Chemistry, 2008, vol. 27, no. 9, pp. 1922–1931.
31 P. C. Ke andM. H. Lamm, "A biophysical perspective of understanding (Nos) at large," Physical Chemistry Chemical Physics, vol. 13, no. 16, pp. 7273–7283, 2011.
32 G. Ghodake, Y. D. Seo, D. Park, and D. S. Lee, Phytotoxicity of carbon nanotubes assessed by brassica juncea and phaseolus mungo, Journal of Nanoelectronics and Optoelectronics, 2010, vol. 5, no. 2, pp. 157–160.
33 Al-Tamimi, H. .; Al-Qaraghouli, A. A. .; Khudair, Z. .; Jbara, A. A. . Effect Of Different Concentrations Of Nano-Magnesium And Nano-Titanium Oxide On The Cumulative Mortality Of Larvae Of Two Species Of Cucurbit Fruit Fly Affiliated With The Dacus In Iraq. JLSAR 2021, 2, 9-13..
34 H. Esaa, F. .; Kassim, J. K. . Identification And Distribution Of Minerals In Soils From Al-Ahrar Area, Waist Province, Iraq. JLSAR 2021, 2, 14-20..
35 J. Muller, F. Huaux, N. Moreau et al., Respiratory toxicity of multiwall carbon nanotubes, Toxicology and Applied, ACS Materials and Interfaces, 2013, vol. 5, no. 5, pp. 1818–1826.
36 P. P. Nadiminti, Y. D. Dong, C. Sayer et al., Nanostructured liquid crystalline particles as an alternative delivery vehicle for plant agrochemicals, ACS Materials and Interfaces, 2013, vol. 5, no. 5, pp. 1818–1826.
37 R. J. Mehta, Y. Zhang, C. Karthik et al., A new class of doped nanobulk high-figure-of-merit thermoelectrics by scalable bottom-up assembly, Nature Materials, , 2012, vol. 11, no. 3, pp.233–240.
38 N. A. Malvadkar, M. J. Hancock, K. Sekeroglu, W. J. Dressick, and M. C. Demirel, An engineered anisotropic nanofilm with unidirectional wetting properties, Nature Materials, , 2010, vol. 9, no. 12, pp. 1023–1028.
39 L. Kong, J. Tang, J. Liu, Y. Wang, L. Wang, and F. Cong, Fluorescent nanoblocks of lanthanide complexes on nano silicon dioxide and carbon nanotube donors with ligand-antenna integration (ALI) structure, Materials Science and Engineering C, 2009, vol. 29, no. 1, pp. 85–91.
40 C. Richard, F. Balavoine, P. Schultz, T. W. Ebbesen, and C. Mioskowski, Supramolecutar self-assembly of lipid derivatives on carbon nanotubes, Science, , 2003, vol. 300, no. 5620, pp. 775–778.
41 C. Lam, J. T. James, R.Mc Cluskey, and R. L.Hunter, Pulmonary toxicity of single-wall carbon nanotubes in mice 7 and 90 days after intractracheal instillation, Toxicological Sciences, , 2004, vol. 77, no. 1, pp. 126–134.
42 H. Qi and T. Hegmann, Impact of nanoscale particles and carbon nanotubes on current and future generations of liquid crystal displays, Journal of Materials Chemistry, 2008 , vol. 18, no. 28, pp. 3288–3294.
43 L. Jinquan, L. Li, C. Hanqing et al., Application of vitamin E to antagonize SWCNTs-induced exacerbation of allergic asthma, Scientific Reports, 2013, vol. 4, article 4275.
44 H. S.Abedalhammed, A. S. Naser, M. H.Al-Maathedy, Th. T.Mohammed, B. T. Jaber, & M. H. Al-Asha'ab, The effect of vitamin e as an antioxidant with different levels of dried tomato pomace supplementation on diets of common carp (cyprinus carpio l) on blood indices. Biochemical and Cellular Archives, 2020, vol 20(2), p. 5173-5176.
45 Guda, M. A., Nasir, A. S., Younus, A. S., & Altamimi, A. J.. Antioxidant enzyme responses of Juncus Aschers.(Et Buch.) Adams to some of environmental stresses and use it as indicators. (2018)EXECUTIVE EDITOR, 9(12), 1102.
46 Guda, M. A., Mutlag, N. H., & Tsear, A. A., December). The use of Atriplex nummularia plant as the hyperaccumulators of silver. In (2020AIP Conference Proceedings (Vol. 2290, No. 1, p. 020041). AIP Publishing LLC.; https://doi.org/10.1063/5.0027565
47 Guda, M. A., Mutlag, N. H., & Alasedi, K. K.). The potential use of Atriplex nummularia plant as contamination indicators of heavy metal in different soils. (2018 Plant Archives, 18(2), 2372-2378.
48 Guda, M. A., Merza, T., & Almayahi, B.. Response of non-enzymatic antioxidants to phragmites Australis (Cav.) Trin. Ex. Steudel Plants of the Environmental Stresses in Baher Alnajaf, (2016) Iraq. Plant Cell Biotech Molec Biol, 17, 140-148.
49 Guda, M. A., Hakeem, J. I., Alabassi, M. M., & Almayahi, B. A.). Effects of Environmental Stress on Nutrients of Typha domingensis Pers. Plant in Najaf, (2017 Iraq. Annual Research & Review in Biology, 1-6. http://journalarrb.com/index.php/ARRB/article/view/26235
50 Guda, M. A., Hakeem, J. I., Alabassi, M. M., & Almayahi, B. A. Phytoremediation of Some Heavy Metals in The Soil of General Company for Tire Industry in Najaf Governorate by Wild Plant Species. (2020). Indian Journal of Environmental Protection, 40(7), 758-763.
51 Guda, M. A., Alkaabiº, Z. S., Albayati, F. S., Alduhaidahawi, F. J., & Almayahi, B. A. Anatomical And Sclerophilic Traits Variation ln Two Varieties Of Olive Plants (Olea europaea L) Growing Under Climate Changes ln Various.season of the year. Indian Journal of Environmental protection,Vol.40,No.1Junuary 20,77-83.
52 Guda, M. A., & Obaid, J. K. The Spatial Variation Study of Spread the Lupus Erythematous Disease in Najaf and Babylon Governorates. (2019). Indian Journal of Forensic Medicine & Toxicology, 13(2).
53 Al-Khafaji, B. A., Guda, M. A., Al-Edhari, A. H., & Altamimi, A. J). Taxonomic Study for the Genus Bupleurum L.(Apiaceae) in Iraq using chloroplast gene RPL16. . (2019 Indian Journal of Public Health, 10(01).
54 Al-Edhari, A. H., Shayma’a, M., Sardar, A. S., Guda, M. A., & Almayahi, B. A.). MORPHOLOGICAL STUDY FOR VALERIANELLA KOTSCHYI BOISS. ANDV. MURICATA (STEV.) BAXT.(VALERIANACEAE) IN KURDISTAN, (2018 IRAQ. Plant Archives, 18(2), 2417-2424.
55 Alduhaidahawi, F. J., Guda, M. A., Al-Graiti, T. A., Abdul-Hussein, A., Al Regawi, S. M., & Al-Edhari, A. H. (2009). Environmental Analysis for Spreading Areas of Scleroderma Disease in Babylon Governorate.
56 Alabassi, M. M., Al Naqeeb, N. A., Guda, M. A., & Jabbar, B. S). The study of drinking water pollution by studying some chemical and biological characteristics in Najaf province, Iraq. . (2020 EurAsian Journal of BioSciences, 14(2), 7159-7164.
57 Adrees, M., Khan, Z. S., Ali, S., Hafeez, M., Khalid, S., ur Rehman, M. Z., ... & Rizwan, M. Simultaneous mitigation of cadmium and drought stress in wheat by soil application of iron nanoparticles. (2020). Chemosphere, 238, 124681.
58 Aebi I.I., (1984). Catalase in vitro. Methods in Enzymology, 105: 121-126.
59 Ahmed, B., Dwivedi, S., Abdin, M. Z., Azam, A., Al-Shaeri, M., Khan, M. S., ... & Musarrat, J. Mitochondrial and chromosomal damage induced by oxidative stress in Zn2+ ions, ZnO-bulk and ZnO-NPs treated Allium cepa roots. (2017). Scientific reports, 7(1), 40685.
60 Ahmed, B., Syed, A., Rizvi, A., Shahid, M., Bahkali, A. H., Khan, M. S., & Musarrat, J. Impact of metal-oxide nanoparticles on growth, physiology and yield of tomato (Solanum lycopersicum L.) modulated by Azotobacter salinestris strain ASM. (2021). Environmental Pollution, 269, 116218.
61 AL-Sultani, Douaa Mahdi Hussein and Guda,Muthik Abd Muslim Biological effects of Zinc Oxide Nanoparticles ZnO (NPs) on Physiological and Anatomical indices of some Plants. .(2022), B.Sc. Ecology–Faculty of Science / University of Kufa,Iraq.
62 Alvim, T. T., & dos Reis Martinez, C. B.. Genotoxic and oxidative damage in the freshwater teleost Prochilodus lineatus exposed to the insecticides lambda-cyhalothrin and imidacloprid alone and in combination. Mutation Research/Genetic (2019)Toxicology and Environmental Mutagenesis, 842, 85-93.
63 Aminot, A., & Rey, F. (2000). Standard procedure for the determination of chlorophyll a by spectroscopic methods. International Council for the Exploration of the Sea, 112, 25.
64 Anjum, S., Anjum, I., Hano, C., & Kousar, S. Advances in nanomaterials as novel elicitors of pharmacologically active plant specialized metabolites: Current status and future outlooks. (2019). RSC advances, 9(69), 40404-40423.
65 Asl, K. R., Hosseini, B., Sharafi, A., & Palazon, J. (2019). Influence of nano‐zinc oxide on tropane alkaloid production, h6h gene transcription and antioxidant enzyme activity in Hyoscyamus reticulatus L. hairy roots. Engineering in Life Sciences, 19(1), 73-89.
66 Bhandari, H. R., Bhanu, A. N., Srivastava, K., Singh, M. N.and Shreya, H. A. Assessment of genetic diversity in crop plants-an overview. (2017). Advances in Plants & Agricultural Research 7: 279-286.
67 Bhavaniramya, S., Vishnupriya, S., Al-Aboody, M. S., Vijayakumar, R., & Baskaran, D. Role of essential oils in food safety: Antimicrobial and antioxidant applications. (2019). Grain & oil science and technology, 2(2), 49-55.
68 bin Hussein, M. Z., Zainal, Z., Yahaya, A. H., & Foo, D. W. V. Controlled release of a plant growth regulator, α-naphthaleneacetate from the lamella of Zn–Al-layered double hydroxide nanocomposite. (2002). Journal of controlled release, 82(2-3), 417-427.
68
69 Breusing, N. ; Grune, T. ; Andrisic, L. ; Atalay, M. ; Bartosz, G. ; Bryan, G. W.; Waldichuk, M.; Pentreath, R. J.; Darracott, Ann "Bioaccumulation of Marine Pollutants [and Discussion]" (2010). Philosophical Transactions of the Royal Society of London. Series Biological Sciences. (1015): 483-505. JSTOR 2418066 .
70 Cakmak, I. Enrichment of cereal grains with zinc: agronomic or genetic biofortification?. (2008). Plant and soil, 302, 1-17.
71 Cakmak, IEnrichment of cereal grains with zinc: agronomic or genetic biofortification?. . (2008). Plant and soil, 302, 1-17.
72 Cavaiuolo, M., Cocetta, G., Spadafora, N. D., Müller, C. T., Rogers, H. J., & Ferrante, A. Gene expression analysis of rocket salad under pre-harvest and postharvest stresses: (2017). A transcriptomic resource for Diplotaxis tenuifolia. PloS one, 12(5), e0178119.
73 Cerda, H., Delincee, H., Haine, H., & Rupp, H. (1997). The DNAcomet assay'as a rapid screening technique to control irradiated food. Mutation Research/Fundamental and Molecular Mechanisms of Mutagenesis, 375(2), 167-181.
74 Chung, S. W., Yu, D. J., & Lee, H. J. Changes in anthocyanidin and anthocyanin pigments in highbush blueberry (Vaccinium corymbosum cv. Bluecrop) fruits during ripening. (2016). Horticulture, Environment, and Biotechnology, 57, 424-430.
75 Copeland, J. Characterizing the Community Dynamics of the Leaf Microbiome. (2014). University of Toronto (Canada).
76 Cruz, J. G., Silveira, T., Richter, V., Wagner, J. G., Neitzke, R. S., Barbieri, R. L., & Vizzotto, M.). Genetic variability of bioactive compounds in Capsicum chinense. (2022Food Science and Technology, 42.
77 Debnath, P., Mondal, A., Sen, K., Mishra, D., & Mondal, N. K. Genotoxicity study of nano Al2O3, TiO2 and ZnO along with UV-B exposure: An Allium cepa root tip assay. (2020). Science of The Total Environment, 713, 136592.
78 Desoky, E. S. M., El-maghraby, L. M., Awad, A. E., Abdo, A. I., Rady, M. M., & Semida, W. M. Fennel and ammi seed extracts modulate antioxidant defence system and alleviate salinity stress in cowpea (Vigna unguiculata). (2020). Scientia Horticulturae, 272, 109576.
79 Dewez D, Goltsev V, Kalaji HM, Oukarroum A Inhibitory effects of silver nanoparticles on photosystem II performance in Lemna gibba probed by chlorophyll fluorescence. (2018) Curr Plant Biol 16:15–21.
80 Dewez, D., Goltsev, V., Kalaji, H. M., & Oukarroum, A. Inhibitory effects of silver nanoparticles on photosystem II performance in Lemna gibba probed by chlorophyll fluorescence. (2018). Current plant biology, 16, 15-21.
81 Dietz, K. J., & Herth, S. Plant nanotoxicologyTrends in plant science, . (2011). 16(11), 582-589.
82 Doolette, C. L., Read, T. L., Li, C., Scheckel, K. G., Donner, E., Kopittke, P. M., ... & Lombi, EFoliar application of zinc sulphate and zinc EDTA to wheat leaves: differences in mobility, distribution, and speciation. . (2018). Journal of Experimental Botany, 69(18), 4469-4481.
83 Duxbury, A.C. and Yentach, C.S.(1956): Plankton pigment monograph.J.Marine Res., 15: 190-191.
84 Elena, M., Katarína, K., Ivana, V., & Zuzana, K). Responses of medicinal plants to abiotic stresses. . (2019In Handbook of Plant and Crop Stress, Fourth Edition (pp. 713-753). CRC Press.
85 Faizan, M., Bhat, J. A., Hessini, K., Yu, F., & Ahmad, P. Zinc oxide nanoparticles alleviates the adverse effects of cadmium stress on Oryza sativa via modulation of the photosynthesis and antioxidant defense system. (2021). Ecotoxicology and Environmental Safety, 220, 112401.
86 García-Gómez, C., Obrador, A., González, D., Babín, M., & Fernández, M. D. Comparative study of the phytotoxicity of ZnO nanoparticles and Zn accumulation in nine crops grown in a calcareous soil and an acidic soil. (2018). Science of the total environment, 644, 770-780.
87 García-López, J. I., Niño-Medina, G., Olivares-Sáenz, E., Lira-Saldivar, R. H., Barriga-Castro, E. D., Vázquez-Alvarado, R., ... & Zavala-García, F. Foliar application of zinc oxide nanoparticles and zinc sulfate boosts the content of bioactive compounds in habanero peppers. (2019). Plants, 8(8), 254.
88 García-López, J. I., Niño-Medina, G., Olivares-Sáenz, E., Lira-Saldivar, R. H., Barriga-Castro, E. D., Vázquez-Alvarado, R., ... & Zavala-García, F. Foliar application of zinc oxide nanoparticles and zinc sulfate boosts the content of bioactive compounds in habanero peppers. (2019). Plants, 8(8), 254.
89 Ghosh, M., Sinha, S., Jothiramajayam, M., Jana, A., Nag, A., & Mukherjee, A. Cyto-genotoxicity and oxidative stress induced by zinc oxide nanoparticle in human lymphocyte cells in vitro and Swiss albino male mice in vivo. (2016). Food and Chemical Toxicology, 97, 286-296.
90 Gichner, T., Znidar, I., Wagner, E. D., & Plewa, M. J. The use of higher plants in the comet assay. (2009). The comet assay in toxicology, 98-119.
91 Gomes, G. P., Constantino, L. V., Corte, L. E. D., Riger, C. J., Chaves, D. S. D. A., & Gonçalves, L. SCharacterization of biochemical compounds and antioxidant activity of "dedo-de-moça" chili pepper accessions. . (2019). Horticultura Brasileira, 37, 429-436.
92 Guda, M. A., Hakeem, J. I., Alabassi, M. M., & Almayahi, B. A. (2020). Phytoremediation of Some Heavy Metals in The Soil of General Company for Tire Industry in Najaf Governorate by Wild Plant Species. Indian Journal of Environmental Protection, 40(7), 758-763.
93 Gupta SD, Agarwal A, Pradhan S (Phytostimulatory effect of silver nanoparticles (AgNPs) on rice seedling growth: an insight from antioxidative enzyme activities and gene expression patterns. 2018)Ecotox Environ Safe 161:624–633.
94 Hassan, R.MKinetics of reduction of Se (IV) by vitamin C with green synthesis of cluster-grapes nanoparticles: . (2022),A Mechanistic approach on electron-transfer of nanoparticle growth rates. J. Mol. Struct. 1250, 131575.
95 Hazeem, L.J.; Waheed, F.A.; Rashdan, S.; Bououdina, M.; Brunet, L.; Slomianny, C.; Boukherroub, R.; Elmeselmani, W.A.,( 2015). Effect of magnetic iron oxide (Fe3O4) nanoparticles on the growth and photosynthetic pigment content of Picochlorum sp. Environ. Sci. Pollut. Res. Int., 22, 11728–11739.
96 Hazeem, L.J.; Waheed, F.A.; Rashdan, S.; Bououdina, M.; Brunet, L.; Slomianny, C. effect of zinc oxide and titanium oxide nanoparticles on growth and chlorophyll a content of Picochlorum sp. (2015). Environmental Science and Pollution Research. 2016;23:2821–2830.
97 Hemeda, H. M., & Klein, B. P. (1990). Effects of naturally occurring antioxidants on peroxidase activity of vegetable extracts. Journal of food Science, 55(1), 184-185.
98 Hernández-Fuentes, A. D., López-Vargas, E. R., Pinedo-Espinoza, J. M., Campos-Montiel, R. G., Valdés-Reyna, J., & Juárez-Maldonado, A. Postharvest behavior of bioactive compounds in tomato fruits treated with Cu nanoparticles and NaCl stress. (2017).Applied Sciences, 7(10), 980.
99 Honda, C., & Moriya, S. Anthocyanin biosynthesis in apple fruit. (2018). The Horticulture Journal, 87(3), 305-314.
100 Jampílek, J., & Kráľová, K. (. Nanobiopesticides in agriculture: State of the art and future opportunities. 2019)Nano-biopesticides today and future perspectives, 397-447.
101 Jampilek, J., & Kralova, K. Potential of nanonutraceuticals in increasing immunity. (2020). Nanomaterials, 10(11), 2224.
102 Jampilek, J.; Kralova, K.; Fedor, P. Bioactivity of nanoformulated synthetic and natural insecticides and their impact on environment. In Nanopesticides; Fraceto, L., de Castro, V.S.S., Grillo, R., Ávila, D., Caixeta Oliveira, H., Lima, R., Eds.; Springer: Cham, Switzerland, 2020; pp. 165–225.
103 Jayasinghe, C., Gotoh, N., Aoki, T., & Wada, S. (. Phenolics composition and antioxidant activity of sweet basil (Ocimum basilicum L.). 2003)Journal of Agricultural and Food chemistry, 51(15), 4442-4449.
104 Jiang, B.; Xu, X.; Mcclements, D.J.; Yuan, B.; Cao, C. Protein corona formation around inorganic nanoparticles: Food plant proteins-TiO2 nanoparticle interactions. (2021). Food Hydrocoll., 115, 106594.
105 Jiraungkoorskul, W. Review of neuro-nutrition used as anti-alzheimer plant, spinach, Spinacia oleracea. (2016). Pharmacognosy reviews, 10(20), 105.
106 K. Van Hoecke, K. A. C. De Schamphelaere, S. Ramirez- Garcia, P. Van der Meeren, G. Smagghe, and C. R. Janssen "Influence of alumina coating on characteristics and effects of SiO2 nanoparticles in algal growth inhibition assays at various pH and organic matter contents, , (2011). Environment International, vol. 37, no. 6, pp. 1118–1125,.
107 K. Yang and Y. Ma, "Computer simulation of the translocation of (Nos) with different shapes across a lipid bilayer, (2010)" Nature Nanotechnology, vol. 5, no. 8, pp. 579–583,.
Received: 15 May 2023/ Accepted: June 10 2023 / Published:15 June 2023
Citation: Owied, O.A.R.; Guda, M.A.M.; Taher, H.I.; Abdulhussein, M.A.A . Plants Anatomical Engineered By Nanomaterials. Revis Bionatura 2023;8 (2) 44. http://dx.doi.org/10.21931/RB/2023.08.02.44