2023.08.02.9
Files > Volume 8 > Vol 8 No 2 2023

The problem associated with tuberculosis in cattle and how this is being addressed
1 Universidad de Concepcion, [email protected].
2 Universidad de Concepción, [email protected].
· *Correspondig author. [email protected]
Available from: http://dx.doi.org/10.21931/RB/2023.08.02.9
ABSTRACT
Bovine tuberculosis (bTB) is a zoonotic disease caused by Mycobacterium bovis that primarily infects cattle but has a wide range of hosts. It represents a global health problem affecting the livestock industry tremendously, with economic losses of about 3 billion annually. Dairy cattle produce a decline of 10% in terms of liters of milk produced and 5% in the meat industry because of live weight loss and seized carcasses in abattoirs. The core of the current control measures implemented in most countries against bTB is based on the diagnosis with tuberculin skin test (TST) and culling of infected animals. Unfortunately, control programs have failed to eradicate the disease since no vaccine protects cattle from infection.
Moreover, the strain of M. bovis Bacillus Calmette Guerin, BCG used as a vaccine for human tuberculosis, interferes with surveillance tools. Nowadays, most researchers have been working on improving the efficacy of BCG through a prime-boost strategy that involves a first immunization with BCG and a booster with different types of vaccines. A less explored approach by experts has been the development of a new vaccine that only includes some protective antigens of M. bovis that should be absent ot in low representation in TST. On the other hand, because TST precludes implementation of Bacille Calmette-Guérin (BCG) vaccine–based control programs, several investigations have been carried out to replace the TST with a DIVA test that allows to differentiation infected from vaccinated animals. In this review, most of the studies cited agree that without an effective vaccine and a compatible diagnosis, no program for eradication would be successful against tuberculosis in cattle.
Keywords: bovine tuberculosis; bTB; Mycobacterium bovis; diagnosis; tuberculin skin test, TST, bPPD; vaccines, DIVA.
INTRODUCTION
Bovine tuberculosis (bTB) is a chronic zoonotic pathology caused by Mycobacterium bovis that belongs to the reportable diseases of the World Organization for Animal Health WOAH (OIE). Mycobacterium bovis may mostly affect cattle, humans, and other domestic and wildlife animals 1 , 2 , 3 . It belongs to the phylum Actinobacteria, family Mycobacteriaceae and genus Mycobacterium 4 ,5 . In the Mycobacterium genus has been identified more than 120 different species, and some of them belong to the Mycobacterium tuberculosis complex (MTC). The most common members of the MTC are M. bovis, M. tuberculosis, M. canettii (oldest strain), M. africanum (responsible for human tuberculosis in Africa), M. pinnipedi (infect seals), M. microti (rodents), and M. caprae (goats and other mammals). M. bovis and M. tuberculosis have 99.95% of genomic homology in their shared genes 6 .
Bovine tuberculosis has a worldwide prevalence of about 13% and can be found in 82 countries around the world 7 . The biggest challenge in those countries is the control of M. bovis in wildlife animals as they act as a reservoir of the pathogen 8 . In all these countries, the disease is associated to tremendous economic losses for the livestock industry, which according to a study performed in USA were estimated in 3 billion dollars per year 9 . Research have shown that bTB generates a fertility loss in females and a decrease in milk production per lactation of 10%. On the other side, the disease also generates losses of 5% in the meat industry since carcasses are seized in slaughterhouses, and there is a live weight loss of animals 10 . It has also been observed that close to 1% of positive bTB cows can develop mastitis 11 . On the other hand, the disease produces high costs in terms of international cattle trading restrictions and dairy and meat by-products to OTF countries 12 . Other costs associated with bTB are control and eradication programs that have as central measures the diagnosis through TST and culling of infected animals to avoid spreading the disease in the herd.
Furthermore, control and eradication programs require removing and replacing infected animals from the pen. Therefore, it causes economic losses not only to farmers but also to the government in terms of indemnity expenses and replacement cost of cattle which can vary from around $500 to 900 USD 13 . The measures included in the control and eradication programs implemented by governments in the countries where bovine tuberculosis is present have shown to be non-enough to prevent infection and eliminate the disease. The need for an effective vaccine that can be applied in cattle and would be authorized by the OIE is still a challenge. Moreover, it is imperative to have a diagnostic tool that can be used with an effective vaccine to support the surveillance strategies. An effective vaccine that strengthens and complements those programs is pivotal in controlling the pathology. Indeed, it has been demonstrated that a strong immunity triggered by a vaccine may defeat M. bovis. The vaccine BCG (Bacillus Calmette-Guérin) used against human tuberculosis but made of Mycobacterium bovis has been trialed before in cattle; however, it has demonstrated a variable range of protection 14 . Currently, most studies are led to improve the effectiveness of BCG in cattle, and in this context, it has been carrying out assays in cows and other animal models. The primary strategy implemented in those studies is based on the prime boost with BCG as a prime and a boost with different types of vaccines, such as subunit recombinant and adenovirus vaccines. Another less explored approach is the development of a new protective vaccine that could include only some immunogenic proteins of M. bovis. These proteins must be included in a low proportion of bPPD of tuberculin so they may not interfere with the TST. On the other side, researchers have also been working on differentiating infected and vaccinated animals and DIVA tests to replace TST because it is incompatible with BCG. The diagnosis of cattle with TST does not allow to discrimination between infected and vaccinated animals since it causes a cross-reaction with the BCG vaccine.
In this article, we seek to review and discuss different studies related to the global problem caused by tuberculosis in cattle. Here it has not only been described the current strategies used in the control of the disease and their drawbacks, but most importantly, we propose an approach that has not been deeply explored as an alternative in developing a new protective vaccine. This could be the study of the most immunogenic proteins of M. bovis that are less representative in bPPD to be included in producing a new vaccine that should not cross-react with the tuberculin skin test.
The Disease In Cattle
In natural conditions, airways are the most frequent route of infection of M. bovis. Using inhalation of the pathogen that is suspended in aerosols, bovines may get infected. Nevertheless, the oral route is the second most common infection in bovines, and they can ingest the bacterium from contaminated water and food 15 . When the microorganism comes from the upper airways, it moves to the pulmonary alveoli. In the alveoli, molecular patterns associated with pathogens are recognized by receptors of innate immune cells, such as macrophages 16 . These cells engulf M. bovis and release chemokines that attract neutrophils and other cells to the infection site, leading to edema with the accumulation of fibrin (inflammatory exudate). Macrophages display the antigen to lymphocytes which then are activated, and as a result, more cells from the immune system reach the site of infection 17 . When immune cells migrate to the site of infection, they accumulate and form tuberculous, which are granulomatous lesions pathognomonic of the disease. In some animals, the
immune system can restrain the initial infection by eliminating the bacterium or inhibiting its multiplication into the tissue 18 . Thus, the microorganism can get into a latency phase in which M. bovis decreases its metabolism to basal levels and remain in a non-replicative state to survive. Nevertheless, the bacterium can reactivate, and the disease will progress, causing a transformation of the granuloma structural organization that in chronic cases, have a necrotic center surrounded by a thick fibrotic tissue layer 19 . The microorganism contained in the granuloma of the lower airways can be transported by phagocytic cells through the lymphatic system until a regional lymph node where a second infection occurs. The primary infection in the lungs, plus the new in the lymph node, integrates the "primary complex" of the disease. When the immune system cannot hold the infection in the primary complex, the pathogen disseminates through the blood (bacteremia) toward other tissues and organs, triggering the generalization of the bTb 20 .
Owing to bTB being a chronic disease with slow progress, most infected animals do not manifest clinical signs in the short term but in months or years after infection. Most animals show unspecific symptoms at the onset of the disease, for example, fluctuating fever, cough, diarrhea, anorexia, weakness, and lymph node inflammation. While acute presentation of bTb is not frequent, it has been observed that the pathology evolves very fast in non-immunocompetent animals, causing death. On the other hand, in animals that overcome the initial infection, the pathology becomes chronic. Some of the clinical signs that infected animals exhibit in advanced stages of the disease are dyspnea, pneumonia, weight loss and emaciation, granulomatous lesions in different organs, and finally ending with the, death 21 . Granulomas can be encountered most frequently in lungs and lymph nodes if M. bovis has come in throughout the respiratory system. Otherwise, granulomas may be in the stomach, spleen, liver or mesenteric lymph nodes if the pathogen penetrates an oral or digestive route. In the inspection, post-mortem is very frequent to find granulomatous lesions in retropharyngeal, mediastinal, and tracheobronchial lymph nodes. Those lesions also commonly affect the lungs (mainly apex zone), stomach, liver, spleen, kidneys, and mammary gland 21 . In severe cases, there is an extrapulmonary presentation of the disease because of the dissemination of M. bovis to the rest of the organism.
Control and prevention of bovine tuberculosis
Despite several countries from European Union, North America, Asia, and Oceania being OTF, the disease has not been fully eradicated. In these countries, bTB is remitted to certain areas, and control and eradication programs against the pathogen's spread are still in force. These programs require huge economic investment because of animal screening and indemnity for culling infected individuals besides other measures such as restrictions in animal movement and surveillance strategies in farms and slaughterhouses.
Detection of M. bovis-infected cattle.
One of the central control measures achieved in the programs against bTB is the identification of infected cattle in the field through the tuberculin skin test TST 22 . The single intradermal tuberculin SIT consists of injecting 0.1 mL of 3000 IU of a bPPD (bovine purified protein derivative), eliciting delayed hypersensitivity response (type IV) mediated by cells when the animal has been previously exposed to the pathogen 23 . This cell immune response is triggered by chemokines and cytokines recruited in the PPD injection site due to previous contact with the microorganism. It has been reported that TST sensitivity range from 57 to 95%, whereas its specificity ranges from 55 to 100% 24 . There are three types of SIT: i) the single caudal fold test (SCFT) which a bPPD is administrated in the tail base, ii) the single intradermal cervical tuberculin (SICT) which a bPPD is injected in the middle of the neck of the animal, iii) the intradermal comparative cervical tuberculin (SICCT) which a bPPD and aPPD (avian purified protein derivative) are applied in two central points of the neck of cattle 25 . In this last, both PPDs are administrated since they allow to discriminate between infections produced by M. bovis and other environmental mycobacteria such as Mycobacterium avium spp paratuberculosis. The tuberculin test results are read 72 hours post-administration and are interpreted according to the skin fold thickness of the inflammatory lesion generated at the injection site. Following the interpretation of the TST, it can be obtained two results: i) negative animals, when was neither observed nor touched any thickness changed at the inoculated site ii) reactor animals, when can be observed and touched a type of edema that maybe go along with blush, warm, and pain at the inoculated site. The intradermal test cannot be performed in animals with an interval of fewer than two months between each application to avoid false positive cases because of the previous sensitization with the PPD 26 .
All suspected or responder animals to the tuberculin test are confirmed through laboratory techniques such as interferon-gamma release assay (IGRAs) and enzyme-linked immunosorbent assay (ELISA).
The IGRA is an in vitro technique that measures a cell-mediated immunity stimulated in response to M. bovis infection. The cytokine IFNγ produced by T lymphocytes of an infected animal is detected in blood samples stimulated with PPD throughout an ELISA plate which is coated with a monoclonal antibody anti- IFNγ. With this assay, infected animals can be identified as soon as two weeks since they were in contact with the pathogen 27 .
On the other side, the ELISA test kit IDDEX is frequently employed in diagnosing infected herds with tuberculosis. However, unlike IGRA, the ELISA is a serological technique that measures antibody immune response against two specific antigens of M. bovis, MPB70 and MPB83, which are immobilized in the plate. Antibodies in sera samples are detected using a secondary conjugated anti-bovine antibody 28 .
Cattle culling and surveillance in slaughterhouses
When a cow is reactive to the tuberculin skin test or confirmed as positive through a laboratory diagnosis, the animal is first isolated and eventually removed from the pen and slaughtered, limiting the risk of continuing to transmit the disease toward the rest of the animals 29 . Culling is one of the control measures mostly used in programs against bovine tuberculosis worldwide. Veterinarians kill the animal in an official abattoir, where the individuals are subjected to an inspection for granulomatous lesions findings 30 . This procedure involves the inspection and incision of lymph nodes in the head (retropharyngeal and tracheobronchial), chest cavity (mediastinal), and organs such as lungs and liver 31 . Samples from these lymph nodes are then sent to an official accredited laboratory for further analysis, such as culture, PCR, and histopathology, to confirm the cases. Once reactive and suspect animal cases are confirmed as positive after organs and carcass inspection, they are included in an official register by the respective government organization 3 . Consequently, in infected farms is performed a sanitation process which provides for quarantine, restriction on animal movement, and the disinfection of premises to remove the disease. After the removal of infected animals and sanitization of the farms, whole herds must periodically be re-evaluated through a tuberculin skin test until the entire herd in negative. These tests should be applied in animals with at least 2 months between and complemented with laboratory analysis 33 , 34. Besides the sanitation and removal strategies, in several countries, the governments provide financial compensation per culled animal to farmers 30 . In England, for example, per animal slaughtered, it is paid about €1500, and in the USA, between $500-2500 US 35 . Because test-and-slaughter approaches are unfeasible, there is an imperative need to develop a new effective vaccine that can support control and eradication programs. Vaccines provide immunity that may protect individuals from pathogens; in reality, they are nearly the most cost-effective solution for controlling infectious diseases.
The vaccine against bTB must meet two main criteria: first, do not interfere with the intradermal tuberculin skin test performed in vivo on animals, and second provide full protection against the Mycobacterium bovis, which is responsible for causing the pathology.
Challenges of vaccination in cattle
Currently, no vaccine is approved by the OIE to be used in cattle against bTB. In the case of human tuberculosis (hTB) produced by Mycobacterium tuberculosis, the strain of M. bovis Bacillus Calmette-Guerin (BCG) is authorized by the World Health Organization (OMS) to be employed as an attenuated vaccine. Despite, both pathologies are closely related in terms of high homology between the microorganisms and similarity in the clinical manifestation of the disease, the use of BCG in cattle for immunization is not allowed 36 . There are two key reasons for this: firstly, BCG is a live attenuated vaccine that includes the whole pathogen; therefore, it shares several antigens with bovine PPD used in the tuberculin skin test or TST 37. This means that TST failed to distinguish BCG-vaccinated cattle from infected ones with a live virulent strain of M. bovis. The interference between BCG and bPPD generates a cellular immune cross-response mediated by memory T cells. These cells generate a recall response when they have been previously exposed to the pathogen, and then animals become positive in TST 38 . Second, BCG has been successfully tested in cattle; however, it has been demonstrated to induce varying levels of protection against M. bovis 39 . The lack of an efficient vaccine against bovine tuberculosis during all these years has provoked the problem caused by the disease has increased due to it still spreading between the species. Hence, it is crucial to have a protective vaccine that complements control and eradication programs executed in most countries where the disease is present. Based on this, in the last 15 years have resurged the interest on the development of a vaccine against bTB that must fulfill all the criteria required to be used in the immunization of cattle.
Requirements must meet a suitable vaccine against bTB
First, an ideal vaccine to bTB must prevent the infection's transmission and establishment, offering whole protection in cattle. Secondly, it must not interfere with the disease's diagnosis and must be cost-effective 39 .
Two main parameters support the effectiveness of a vaccine against bovine tuberculosis. Firstly, the level of lessons in pathognomonic organs and tissues directly relates to bacterial load after a challenge with a virulent strain of M. bovis. In the second place, the immune response triggered by the vaccine is determined by different subsets of T cells and antibodies 40 .
It has been necessary to establish a set of immunological markers, predictors and correlatives of a protective response toward M. bovis. Thanks to different studies performed on human and bovine tuberculosis, it has been discovered essential attributes are involved in protective response against virulent strains of the pathogen 41 . A protective vaccine must lead to a robust innate response, the first line of protection. Specialized antigen-presenting cells (APCs) like dendritic cells (CDs), macrophages, natural killer (NK) cells and gamma delta T (γδ T) cells play a crucial role then for stimulating proper adaptive immunity 42 .
If the vaccine can induce a solid initial innate response, it can later trigger an early and potent adaptive immunity mediated by T CD4+ and CD8+ lymphocytes. The adaptive response through T CD4+ lymphocytes it should be Th1 type, characterized by the expression of proinflammatory cytokines such as IFNγ, TNFα, IL12 e IL2 43 . The vaccine must induce high levels of IFNγ produced by memory and effector T cells. In several studies, IFNγ has been associated with a protective immune response against M. bovis and its role in activating effector T lymphocytes and macrophages responsible for killing the mycobacteria. On the other side, it has also been described that IFNγ stimulates cytotoxic cells capable of destroying infected cells. An ideal vaccine must also induce a proper memory cell response that can answer faster and competently in reinfection cases44 .
Furthermore, the vaccine should stimulate polyfunctional cells that express the surface markers CD44hi, CD45RO+, and CD62Lo, and have the capacity of simultaneously secrete IFNγ, TNFα, and IL2 45 . On the other hand, it has been demonstrated that cell immune response type Th17 also plays a crucial role in protection against M. bovis during the initial establishment of the infection. This Th17 response is specific toward some antigens of the microorganism, and it is mediated by the cytokines IL17, IL21, e IL22. The action of Th17 cells and the respective cytokines have been negatively correlated with the development of the pathology and positively with protection against the disease in cattle 46 . The level of security a vaccine against tuberculosis provides is measured according to bacterial loading associated with the number of colony-forming units (CFU).
Consequently, in vaccinated groups of animals, an ideal vaccine must avoid or reduce the colonization of the microorganism in the different organs. This last means that the vaccine must inhibit the establishment of the infection in comparison with animal control groups that have not been received vaccinated. Figure 1 briefly displayed the main cell type, signaling pathways activated and cytokines pattern that is expected to be involved in the innate and adaptive immune response after vaccination of cattle with a protective vaccine.
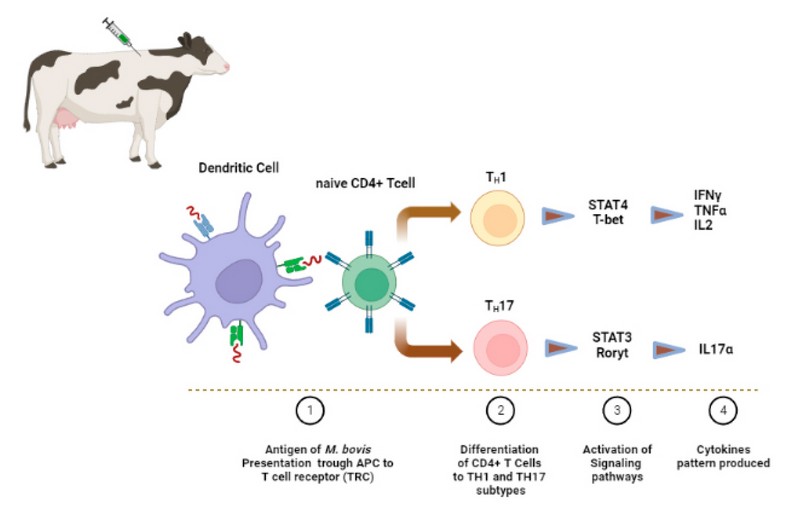
Figure 1. Expected immune response triggered by a protective vaccine against tuberculosis in cattle. The microorganism or antigens of M. bovis inoculated through a vaccine are first recognized by phagocytic cells that ingest the pathogen. This is processed inside of specialized vesicles containing different types of enzymes. Then, the antigens are exposed through histocompatibility molecules (MHC) located on the surface of antigen-presenting cells (APC) to naïve lymphocytes. A protective vaccine against Mycobacterium bovis should mainly activate CD4+ T cells and prompt its differentiation into T helper Th1 and Th17 subsets. Specific signaling pathways must be activated in both responses, the STAT4 and the STAT3, for the expression of the transcription factor T-bet and Roryt since they are responsible for the proinflammatory cytokines profile related to protective responses that involve INFγ, TNFα, IL2 e IL17, respectively.
The second requirement that a bTB-suitable vaccine candidate must meet is not to sensitize animals to react with the TST, which is used as the main tool for cattle diagnosis. This cross-reactivity is in response to immunogenic proteins of Mycobacterium bovis, which are components of bovine PPD (bPPD) of tuberculin skin test. Therefore, when an animal is immunized with a vaccine that shares the same antigens as the TST, as is the case of the live attenuated BCG when TST is performed, the animal will develop a local cellular response in the site of injection. This, hinder differentiating vaccinated from infected animals with a pathogenic strain 47 . One approximation for this problem may consider the development of new protective vaccines that only include some antigens from M. bovis that would be absent or in low proportion in the bPPD of the tuberculin. This list is based on the theory that as bPPD is a cocktail of numerous proteins, the immune response of the antigens that are in low amounts can be waned by the others that are in high doses. According to this, different studies have described the composition of the bPPD; however, none provide full knowledge of the protein conformation. A well-defined understanding of bPPD composition can contribute to identifying and selecting suitable candidate proteins for developing a new vaccine. It is important to note that has been observed some discrepancies in terms of protein components of different PPD preparations. Currently, there are different research that has studied the PPD composition. Among them stand out, a study performed by Rberto et al. 2017 compared the composition of four other preparations of bPPD from the AN5 strain. One of the bPPD was from Spain, two from Italy and the last one from the Netherlands. Proteins from each bPPD were precipitated by TCA and analyzed through bottom-up proteomics and then by mass spectrometry. In this work, 356 proteins were identified; 85% were found to be shared among the four bPPD preparations. From the total, 198 proteins were detected for the first time; 19 proteins were concordant with previously analyzed bPPD proteomes from the United Kingdom and Brazil, and 78 with bPPD from Korea. In addition, 78 proteins were found in common with the United Kingdom, Brazilian and Korean bPPDs. According to the same research, the most abundant proteins in all bPPD preparations are ten: 1) ESAT-6-like protein EsxB, 2) 6 kDa early secretory antigenic target, 3) Immunogenic protein MPB70, 4) ESAT-6-like protein EsxN, 5) 14 kDa antigen, 6) Meromycolate extension acyl carrier protein, 7)10 kDa chaperonin, 8) GroES protein, 9) Immunogenic protein MPB63, and 10) 50S ribosomal protein L7/L12.
Another research carried out by Borsuk et al., 2009 , compared two bovine and two avium PPD preparation from Brazil and UK. This study used the microcolumn reversed-phase liquid chromatography-electrospray ionization tandem mass spectrometry (LC/MS/MS). This method allows the separation of proteins through HPLC and their detection by a mass spectrometer. In total, 171 proteins were discovered among the four bPPD. Among the Brazilian and UK bovine PPD was found 37 common proteins, 11 were unique in Brazilian bPPD and 52 to UK bPPD. On the other hand, 21 proteins were present exclusively in the bovine PPDs but were not identified in the avium PPDs. According to this work, in the Brazilian bPPD it was observed that the most abundant proteins were elongation factor EF-Tu – Rv0685 and Rv0865 (probable molyb- dopterin biosynthesis Mog protein), whereas in the UK bPPDs the most abundant proteins were EsxB protein (ESAT-6 like protein EsxB – Rv3874) and the 10 kDa chaperone protein (Rv3418c).
A second approach to the problem of the cross-reaction between bTB vaccine and TST is the development of differentiating infected from vaccinated animals (DIVA) tests. These assays may replace the tuberculin skin test, and heterologous prime boost strategies with BCG can be used as effective immunization. In order to develop a DIVA test to diagnose bovine tuberculosis in animals, it is necessary first to identify potential protein candidates. The two antigens well-characterized from M. tuberculosis and M. bovis ESAT-6 and CFP-10 have been tested as putative DIVA candidates. Both proteins are encoded in the RD1 region of the genome of the Mycobacterium, which is absent in BCG strains because of its attenuation process. As the RD1 locus is not in the genome of no BCG, neither ESAT-6 nor CFP-10 would be expressed in the strain. Thus, these antigens should not elicit a cross reactivity when animals are vaccinated with BCG. In fact, in a study, ESTA-6 and CFP-10 have been used as diagnostic proteins that can differentiate infected from vaccinated animals.
On the other hand, the bPPD applied in animals during the same study showed that over 70% of BCG-vaccinated cattle reacted to the TST 50 . In another work performed in Chile, researchers from University of Chile implemented and validated an ELISA DIVA assay using the proteins ESAT-6, CFP-10 and Rv3615. Based on this study, it was found that the test was effective in detecting infected animals with M. bovis, yet it is inefficient to test many samples because it is costly and slow 51 . More studies are required to discover new DIVA antigens that can be used in diagnosing bTB. Figure 2 summarizes two potential approaches mentioned in this article to develop a strategy that could allow the use of a compatible vaccine with a diagnosis in bovine tuberculosis.
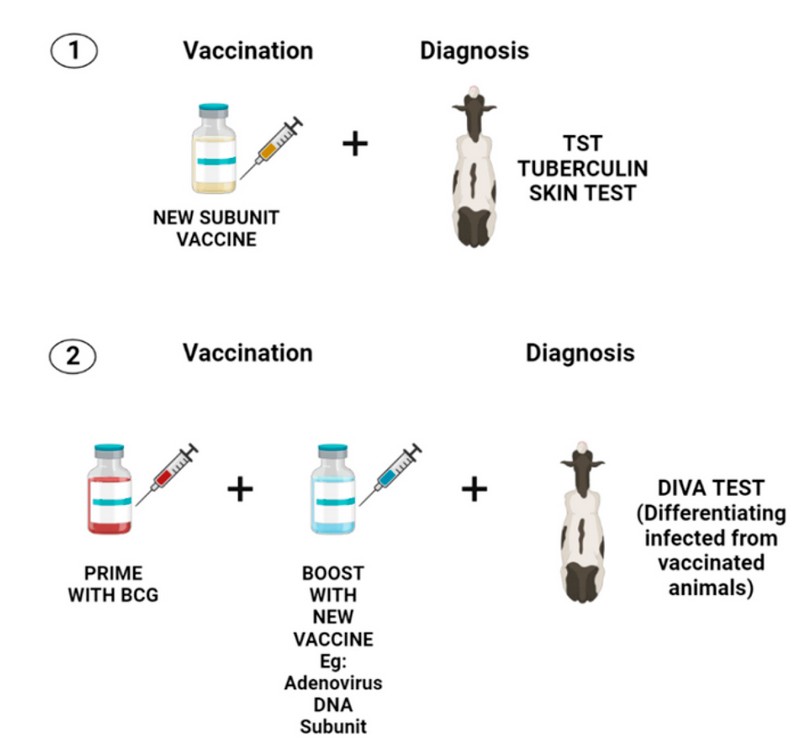
Figure 2. Two potential approaches for consistent vaccination and diagnosis in bovine tuberculosis. The first approach (1) involves the development of a new subunit vaccine that should include the most immunogenic proteins of M. bovis. Those proteins must be absent or in a lower proportion in bPPD to diminished cross-reactivity with TST. This first approach seems to be less studied because only a few reports describe the protein composition of bPPD. In addition, it entails the development of a new vaccine that must demonstrate to elicit a better immune response than BCG. The second approach (2) requires a heterologous prime boost using BCG and a new vaccine that must improve the immunity offered by BCG. Furthermore, this vaccination strategy needs the development of a DIVA diagnosis that only includes antigens that are missing in BCG to avoid cross-reactivity with the vaccine.
New strategies of vaccination.
Thus far, there is not an approved vaccine by the OIE against bovine tuberculosis. On the contrary, for human tuberculosis caused by Mycobacterium tuberculosis, the Bacillus Calmette-Guérin, BCG is allowed, which is a live attenuated vaccine produced from M. bovis. BCG is administrated in infants all around the world as an immunization routine for M. tuberculosis 52 . Although M. tuberculosis and M. bovis are closely related regarding genomic homology and disease presentation, BCG can not be used to prevent bTB since it has low effectiveness and interferes with the major surveillance tool, the tuberculin skin test 53 .
Because bTB tremendously impacts the livestock industry and public health, in the last decades have emerged high interest in developing new effective vaccines against the disease. Researchers have been studying different type of vaccines in animal models to assess their ability to protect against a challenge with M. tuberculosis and M. bovis. The approaches in the development of human tuberculosis vaccines could also be applied for bovine tuberculosis as M. bovis and M. tuberculosis share high genomic homology, and thus, the mechanism of both pathogens in causing the disease is very alike. Therefore, it is important to consider human tuberculosis advances in terms of studying how to face the problem of tuberculosis in cattle caused by M. bovis.
Nowadays, the efforts are mostly focused on vaccination strategies that increase BCG efficacy. These involve a heterologous prime boost with BCG as a prime and recombinant BCG (rBCG) 54,55 , subunit proteins 56 , DNA vaccines 57 , or viral vector vaccines 55 as a boost. Next, we described some of these vaccines and those currently under clinical trials (Table 1).
Recombinant BCG includes strains producing Th1 cytokines to augment the efficacy of wild-type BCG. One type of this rBCG is rBCG::IL-2, that shown to enhance the T-cell and antibody immune response in mice after immunization, proving its greater immunomodulatory effects compared with regular BCG 58 . There are rBCG strains producing non-bacterial immunomodulatory proteins, such as rBCG::Flt3L. The Fms-like tyrosine kinase 3 ligand (Ftl3L) promoted APCs and was over-produced in an rBCG strain. Mice vaccinated with rBCG::Flt3L exhibited more robust IFN-γ responses in the spleen and draining lymph nodes contrasted to wild-type BCG. The recombinant strain was also safer than the parental strain in immune-deficient mice 59 . Other rBCG are strained, over-producing high immunogenic M. bovis proteins like Ag85b. Vaccination with rBCG::Ag85b has shown in mice a lessing of the lessons in lungs compared to immunized groups with the wild-type BCG 54 . Another example of recombinant BCG is the one that lacks of zmp1gene, associated with hindering phagolysosome fusion in infected macrophages. It was reported that this vaccine induce a more robust T-cell response characterized by a rise in the secretion of IFNγ compared with the wild-type BCG 60 .
Several protein subunit vaccines have been tested alone or co-administrated with BCG in animals under experimental conditions. These vaccines have demonstrated its capacity to induce a protective response by itself or boost BCG immunity against virulent M. bovis and M. tuberculosis challenge. In a study, culture filtrate proteins or CFP from M. bovis in combination with bovine IL-2 were used to immunize calves of 6 months old to assesses the effectiveness of the vaccine for stimulating a protective immune response against an intratracheal challenge. The results showed that the vaccine prompted a strong cellular and antibody response significantly reducing the degree of lung lesions in comparison with the control group 61 .
In another investigation, a protein subunit vaccine known as H107 that included antigens from M. tuberculosis (PPE68, ESAT-6, EspI, EspC, and EspA, MPT64, MPT70, and MPT83) was tried. The analysis performed with the vaccine in a murine model disclosed that all chosen antigens induced substantial protection except for MPT64. Protein ESAT-6 was the most protective antigen in mice, followed by EspI, EspA, and EspC. Furthermore, it was found that combining BCG with H107 increases the immunity triggered by BCG alone, characterized by an expansion of the CD4 T cells and a Th17 cell response 62 . Recently, a group of researchers have been developing a new subunit vaccine based on highly antigenic fusion proteins from M. bovis and M. tuberculosis. This is H65, composed of six ESX-secreted antigens EsxD, EsxC, ExsG, EsxH, ExsW and EsxV formulated in CAF01 liposomal adjuvant. According to this, it was shown that in the M. bovis mouse model of infection the administration of BCG plus H65 was much more protective than H65 alone 63 . In addition to protein subunit vaccines, DNA vaccines have been developed that encode specific antigens of M. bovis. An example is a DNA-E6 that encodes the protein ESAT-6, which is absent in BCG strains. Researchers immunized groups of BALB/c mice with DNA-E6 alone and co-administrated with BCG, and afterward, the animals were challenged with low-dose virulent M. tuberculosis H37Rv. This study found that the administration of DNA-E6 and BCG induced more secretion of IFNγ and less pathological changes in lung and spleen than the DNA-E6 alone 64 . In another study, a DNA vaccine that encodes for three mycobacterial antigens Rv3407, Ag85A, and HspX was tested in a murine model. The vaccine triggered an antigen-specific cellular and humoral immune response as well as conferred protection against a challenge performed with M. Tuberculosis aerosol 65 .
Moreover, adenoviruses vaccines boosting BCG have been tried. Among these is MVA85A, which corresponds to a modified vaccine from a recombinant attenuated Ankara virus engineered to express Ag85A from M. tuberculosis. Individuals immunized with a prime-boost BCG- MVA85A showed high antigen 85A-specific CD8+ T cells after being boosted with MVA85A 66 . Another adenovirus vaccine used to boost BCG is Ad85A, an attenuated recombinant vaccine based on the human adenovirus type 5 (AdHu5), which was also developed to express Ag85A. It was found that Ad85A stimulates polyfunctional CD4+ and CD8+ T cell immunity in previously BCG-vaccinated individuals 67 .
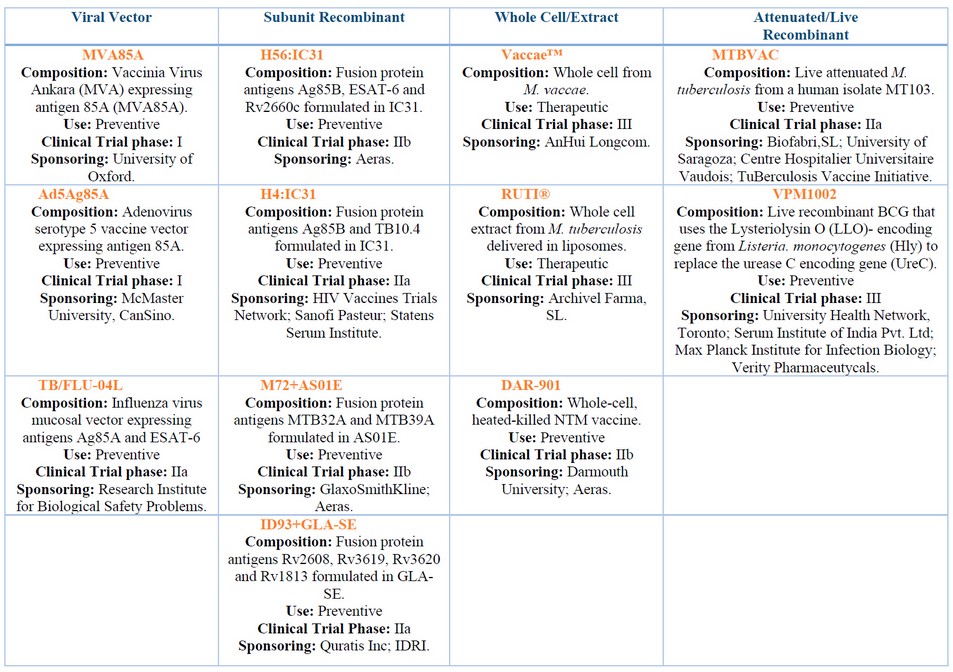
Table 1. Shows several vaccine candidates currently under clinical trials and were classified according to its composition. The information for all these vaccines was retrieved from the following website https://clinicaltrials.gov/.
CONCLUSIONS
This article briefly describes the enormous damage caused by Mycobacterium bovis in the livestock industry and gives an approach to its possible solutions. According to the studies reviewed, all of them agree that without an effective vaccine and a compatible diagnosis, no program for eradication would be victorious against tuberculosis in cattle. In these terms, based on the scientific information gathered, two approaches could offer a true solution for bovine tuberculosis. The first one is the development of a new protective vaccine that precludes in the near future the use of BCG in animals, so it may be used with TST. This vaccine should comprise some of the most immunogenic proteins from M. bovis to protect against de pathogens.
Moreover, those selected proteins should be less representative in bPPD of tuberculin, hence may not cause a cross-reaction with the diagnosis. However, we noticed that only few studies describe the protein composition of bPPD. For this purpose, it is essential a deep knowledge of all protein components of bPPD. The second one is the replacement of TST with a DIVA test which also involves a strategy of vaccination that allows to the improvement of the effectiveness of BCG. Then finally, BCG would be used in cattle. The DIVA tests consider M. bovis proteins that are not expressed in BCG (e.g.: ESAT6/CFP10), so unlikely could occur a cross-reaction between the vaccine and the surveillance tool.
On the other hand, BCG enhancement consists of a prime-boost where the immune system is first primed with a dose of BCG, and later it is boosted with a subunit vaccine. This last approach seems to be more explored than the first one because more studies about it are available, and it was easier to incorporate them in our review. In brief, even though there is a lot of evidence in the literature that prime-boost with BCG and other types of vaccines gives better results in terms of protection than administrating BCG by itself, it needs to be compatible with an efficient diagnosis of the disease. Therefore, a definitive solution for tuberculosis in cattle would not be accomplished in the short term.
Conflicts of Interest: "The authors declare no conflict of interest."
REFERENCES
1. O'reilly LM, Daborn CJ. The epidemiology of Mycobacterium bovis infections in animals and man: a review. Vol. 1, Tubercle and Lung Disease. 1995.
2. de La Rua-Domenech R. Human Mycobacterium bovis infection in the United Kingdom: Incidence, risks, control measures and review of the zoonotic aspects of bovine tuberculosis. Vol. 86, Tuberculosis. 2006. p. 77–109.
3. Walker TM, Ip CLC, Harrell RH, Evans JT, Kapatai G, Dedicoat MJ, et al. Whole-genome sequencing to delineate Mycobacterium tuberculosis outbreaks: A retrospective observational study. Lancet Infect Dis. 2013 Feb;13(2):137–46.
4. Tibesso G. Review on epidemiological features of Mycobaterium bovis at the human, cattle and wildlife interface in Ethiopia. Biom Biostat Int J. 2018 Sep 28;7(5).
5. Integrated Taxonomic Information System - Report (ITIS). 2022.
6. Forrellad MA, Klepp LI, Gioffré A, García JS, Morbidoni HR, de la Paz Santangelo M, et al. Virulence factors of the mycobacterium tuberculosis complex. Vol. 4, Virulence. Taylor and Francis Inc.; 2013. p. 3–66.
7. World Organization for Animal Health (WHOA). Bovine Tuberculosis [Internet]. 2022 [cited 2023 Feb 26]. Available from: https://www.woah.org/en/disease/bovine-tuberculosis/
8. The European Union One Health 2019 Zoonoses Report. EFSA Journal. 2021 Feb 1;19(2).
9. Olmstead AL, Rhode PW. An impossible undertaking: The eradication of bovine tuberculosis in the United States. Journal of Economic History. 2004;64(3):734–72.
10. Tschopp R, Zinsstag J, Conlan A, Gemechu G, Wood J. Productivity loss and cost of bovine tuberculosis for the dairy livestock sector in Ethiopia. Prev Vet Med. 2022 May 1;202.
11. Bolaños CAD, de Paula CL, Guerra ST, Franco MMJ, Ribeiro MG. Diagnosis of mycobacteria in bovine milk: An overview. Vol. 59, Revista do Instituto de Medicina Tropical de Sao Paulo. Instituto de Medicina Tropical de Sao Paulo; 2017.
12. Phoenix JH. Trading with risk: associating bovine tuberculosis to cattle commodities in risk-based trading. J Cult Econ. 2021;14(3):293–305.
13. Smith RL, Tauer LW, Sanderson MW, Gröhn YT. Minimum cost to control bovine tuberculosis in cow-calf herds. Prev Vet Med. 2014 Jul 1;115(1–2):18–28.
14. Ábalos P, Valdivieso N, de Val BP, Vordermeier M, Benavides MB, Alegría-Morán R, et al. Vaccination of Calves with the Mycobacterium bovis BCG Strain Induces Protection against Bovine Tuberculosis in Dairy Herds under a Natural Transmission Setting. Animals. 2022 May 1;12(9).
15. Cassidy JP. The pathogenesis and pathology of bovine tuberculosis with insights from studies of tuberculosis in humans and laboratory animal models. In: Veterinary Microbiology. 2006. p. 151–61.
16. Domingo M, Vidal E, Marco A. Pathology of bovine tuberculosis. Res Vet Sci. 2014;97(S):S20–9.
17. Neill SD, Pollock JM, Bryson DB, Hanna J. Pathogenesis of Mycobacterium bovis infection in cattle. Vol. 40, Veterinary Microbiology. 1994.
18. Sakamoto K. The Pathology of Mycobacterium tuberculosis Infection. Vol. 49, Veterinary Pathology. 2012. p. 423–39.
19. Gengenbacher M, Kaufmann SHE. Mycobacterium tuberculosis: Success through dormancy. Vol. 36, FEMS Microbiology Reviews. 2012. p. 514–32.
20. Heemskerk D, Caws M, Marais B, et al. Clinical Manifestations. In: Tuberculosis in adults and children. London: Springer; 2015.
21. Ayele WY, Neill SD, Zinsstag J, Weiss MG, Pavlik I. Bovine tuberculosis: an old disease but a new threat to Africa. Vol. 8, INT J TUBERC LUNG DIS. 2004.
22. Awah-Ndukum J, Temwa J, Ngwa VN, Mouiche MM, Iyawa D, Zoli PA. Interpretation criteria for comparative intradermal tuberculin test for diagnosis of bovine tuberculosis in cattle in Maroua Area of Cameroon. Vet Med Int. 2016;2016.
23. Bayissa B, Sirak A, Zewude A, Worku A, Gumi B, Berg S, et al. Field evaluation of specific mycobacterial protein-based skin test for the differentiation of Mycobacterium bovis-infected and Bacillus Calmette Guerin-vaccinated crossbred cattle in Ethiopia. Transbound Emerg Dis. 2022 Jul 1;69(4):e1–9.
24. Doan TN, Eisen DP, Rose MT, Slack A, Stearnes G, McBryde ES. Interferon-gamma release assay for the diagnosis of latent tuberculosis infection: A latent-class analysis. PLoS One. 2017 Nov 1;12(11).
25. Good M, Clegg TA, Costello E, More SJ. The comparative performance of the single intradermal test and the single intradermal comparative tuberculin test in Irish cattle, using tuberculin PPD combinations of differing potencies. Veterinary Journal. 2011 Nov;190(2).
26. Monaghan A' ML, Doherty ML, Collins JD, Kazda JF, Quinn PJ. The tuberculin test. Vol. 40, Veterinary Microbiology. 1994.
27. Sadatsafavi M, Shahidi N, Marra F, FitzGerald MJ, Elwood KR, Guo N, et al. A statistical method was used for the meta-analysis of tests for latent TB in the absence of a gold standard, combining random-effect and latent-class methods to estimate test accuracy. J Clin Epidemiol. 2010 Mar;63(3):257–69.
28. Filho PMS, Ramalho AK, Silva AM, Issa MA, Mota PMPC, Silva CHO, et al. Diagnostic performance of a commercial ELISA used as a complementary test for bovine tuberculosis in two bovine herds with different disease status. Arq Bras Med Vet Zootec. 2020 Jan 1;72(1):1–8.
29. Donnelly CA, Woodroffe R, Cox DR, Bourne FJ, Cheeseman CL, Clifton-Hadley RS, et al. Positive and negative effects of widespread badger culling on tuberculosis in cattle. Nature. 2006 Feb 16;439(7078):843–6.
30. Arnot LF, Michel A. Challenges for controlling bovine tuberculosis in south africa. Vol. 87, Onderstepoort Journal of Veterinary Research. AOSIS (pty) Ltd; 2020.
31. Woldemariyam FT, Markos T, Shegu D, Abdi KD, Paeshuyse J. Evaluation of postmortem inspection procedures to diagnose bovine tuberculosis at debre birhan municipal abattoir. Animals. 2021 Sep 1;11(9).
32. Asseged B, Woldesenbet Z, Yimer E, Lemma E. Evaluation of Abattoir Inspection for the Diagnosis of Mycobacterium bovis Infection in Cattle at Addis Ababa Abattoir. 2004.
33. Smith RL, Tauer LW, Schukken YH, Lu Z, Grohn YT. Minimization of bovine tuberculosis control costs in US dairy herds. Prev Vet Med. 2013 Nov 1;112(3–4):266–75.
34. Che-Amat A, Risalde MÁ, González-Barrio D, Ortíz JA, Gortázar C. Effects of repeated comparative intradermal tuberculin testing on test results: A longitudinal study in TB-free red deer. BMC Vet Res. 2016 Sep 5;12(1).
35. Smith RL, Tauer LW, Schukken YH, Lu Z, Grohn YT. Minimization of bovine tuberculosis control costs in US dairy herds. Prev Vet Med. 2013 Nov 1;112(3–4):266–75.
36. Milián-Suazo F, González-Ruiz S, Contreras-Magallanes YG, Sosa-Gallegos SL, Bárcenas-Reyes I, Cantó-Alarcón GJ, et al. Vaccination Strategies in a Potential Use of the Vaccine against Bovine Tuberculosis in Infected Herds. Vol. 12, Animals. MDPI; 2022.
37. Chandran A, Williams K, Mendum T, Stewart G, Clark S, Zadi S, et al. Development of a diagnostic compatible BCG vaccine against Bovine tuberculosis. Sci Rep. 2019 Dec 1;9(1).
38. Chambers MA, Carter SP, Wilson GJ, Jones G, Brown E, Hewinson RG, et al. Vaccination against tuberculosis in badgers and cattle: An overview of the challenges, developments and current research priorities in Great Britain. Vol. 175, Veterinary Record. British Veterinary Association; 2014. p. 90–6.
39. Balseiro A, Thomas J, Gortázar C, Risalde MA. Development and challenges in animal tuberculosis vaccination. Vol. 9, Pathogens. MDPI AG; 2020. p. 1–31.
40. Garrido JM, Sevilla IA, Beltrán-Beck B, Minguijón E, Ballesteros C, Galindo RC, et al. Protection against tuberculosis in eurasian wild boar vaccinated with heat-inactivated mycobacterium bovis. PLoS One. 2011 Sep 14;6(9).
41. Thomas J, Risalde MÁ, Serrano M, Sevilla I, Geijo M, Ortíz JA, et al. The response of red deer to oral administration of heat-inactivated Mycobacterium bovis and challenge with a field strain. Vet Microbiol. 2017 Sep 1;208:195–202.
42. Blanco FC, Gravisaco MJ, Bigi MM, García EA, Marquez C, McNeil M, et al. Identifying Bacterial and Host Factors Involved in the Interaction of Mycobacterium bovis with the Bovine Innate Immune Cells. Front Immunol. 2021 Jul 15;12.
43. Flesch IEA, Kaufmann SHE. Role of Cytokines in Tuberculosis. Immunobiology. 1993;189(3–4):316–39.
44. Rhodes SG, Palmer N, Graham SP, Bianco AE, Hewinson RG, Vordermeier HM. Distinct Response Kinetics of Gamma Interferon and Interleukin-4 in Bovine Tuberculosis. Vol. 68, INFECTION AND IMMUNITY. 2000.
45. Lewinsohn DA, Lewinsohn DM, Scriba TJ. Polyfunctional CD4+ T cells as targets for tuberculosis vaccination. Vol. 8, Frontiers in Immunology. Frontiers Media SA; 2017.
46. Lyadova I v., Panteleev A v. Th1 and Th17 Cells in Tuberculosis: Protection, Pathology, and Biomarkers. Mediators Inflamm. 2015;2015.
47. Buddle BM, Vordermeier HM, Chambers MA, de Klerk-Lorist LM. Efficacy and safety of BCG vaccine for control of tuberculosis in domestic livestock and wildlife. Vol. 5, Frontiers in Veterinary Science. Frontiers Media SA; 2018.
48. Roperto S, Varano M, Russo V, Lucà R, Cagiola M, Gaspari M, et al. Proteomic analysis of protein purified derivative of Mycobacterium bovis. J Transl Med. 2017 Apr 3;15(1).
49. Borsuk S, Newcombe J, Mendum TA, Dellagostin OA, McFadden J. Identification of proteins from tuberculin purified protein derivative (PPD) by LC-MS/MS. Tuberculosis. 2009 Nov;89(6):423–30.
50. Vordermeier HM, Whelan A, Cockle PJ, Farrant L, Palmer N, Hewinson RG. Use of synthetic peptides derived from the antigens ESAT-6 and CFP-10 for differential diagnosis of bovine tuberculosis in cattle. Clin Diagn Lab Immunol. 2001;8(3):571–8.
51. VASQUES ILLANES MN. IMPLEMENTACIÓN Y VALIDACIÓN DE UNA PRUEBA SANGUÍNEA DIVA CON ANTÍGENOS ESAT-6, CFP-10 Y Rv3615c PARA EL DIAGNÓSTICO DE TUBERCULOSIS BOVINA EN CHILE. [SANTIAGO]: UNIVERSIDAD DE CHILE; 2018.
52. World Organization for animal health. Bovine Tuberculosis. 2022.
53. Whelan AO, Coad M, Upadhyay BL, Clifford DJ, Hewinson RG, Vordermeier HM. Lack of correlation between bcg-induced tuberculin skin test sensitisation and protective immunity in cattle. Vaccine. 2011 Jul 26;29(33):5453–8.
54. Yuan X, Teng X, Jing Y, Ma J, Tian M, Yu Q, et al. A live attenuated BCG vaccine overexpressing multistage antigens Ag85B and HspX provides superior protection against Mycobacterium tuberculosis infection. Appl Microbiol Biotechnol. 2015 Dec 1;99(24):10587–95.
55. Khan A, Sayedahmed EE, Singh VK, Mishra A, Dorta-Estremera S, Nookala S, et al. A recombinant bovine adenoviral mucosal vaccine expressing mycobacterial antigen-85B generates robust protection against tuberculosis in mice. Cell Rep Med. 2021 Aug 17;2(8).
56. Santema W, Hensen S, Rutten V, Koets A. Heat shock protein 70 subunit vaccination against bovine paratuberculosis does not interfere with current immunodiagnostic assays for bovine tuberculosis. Vaccine. 2009 Apr 14;27(17):2312–9.
57. Skinner MA, Buddle BM, Wedlock DN, Keen D, De Lisle GW, Tascon RE, et al. A DNA prime-Mycobacterium bovis BCG boost vaccination strategy for cattle induces protection against bovine tuberculosis? Infect Immun. 2003 Sep 1;71(9):4901–7.
58. Young S, O'Donnell M, Lockhart E, Buddle B, Slobbe L, Luo Y, et al. Manipulation of immune responses to Mycobacterium bovis by vaccination with IL-2- and IL-18-secreting recombinant bacillus Calmette Guerin. Immunol Cell Biol. 2002;80(3):209–15.
59. Triccas JA, Shklovskaya E, Spratt J, Ryan AA, Palendira U, Barbara BF, et al. Effects of DNA- and Mycobacterium bovis BCG-based delivery of the Flt3 Ligand on protective immunity to Mycobacterium tuberculosis. Infect Immun. 2007 Nov;75(11):5368–75.
60. Johansen P, Fettelschoss A, Amstutz B, Selchow P, Waeckerle-Men Y, Keller P, et al. Relief from Zmp1-mediated arrest of phagosome maturation is associated with facilitated presentation and enhanced immunogenicity of mycobacterial antigens. Clinical and Vaccine Immunology. 2011 Jun;18(6):907–13.
61. Wedlock DN, Vesosky B, Skinner MA, De Lisle GW, Orme IM, Buddle BM. Vaccination of Cattle with Mycobacterium bovis Culture Filtrate Proteins and Interleukin-2 for Protection against Bovine Tuberculosis. Vol. 68, INFECTION AND IMMUNITY. 2000.
62. Woodworth JS, Clemmensen HS, Battey H, Dijkman K, Lindenstrøm T, Laureano RS, et al. A Mycobacterium tuberculosis-specific subunit vaccine that provides synergistic immunity upon co-administration with Bacillus Calmette-Guérin. Nat Commun. 2021 Dec 1;12(1).
63. Blanco FC, García EA, Aagaard C, Bigi F. The subunit vaccine H65 + CAF01 increased the BCG- protection against Mycobacterium bovis infection in a mouse model of bovine tuberculosis. Res Vet Sci. 2021 May 1;136:595–7.
64. Fan X, Gao Q, Fu R. DNA vaccine encoding ESAT-6 enhances the protective efficacy of BCG against Mycobacterium tuberculosis infection in mice. Scand J Immunol. 2007 Nov;66(5):523–8.
65. Mir FA, Kaufmann SHE, Eddine AN. A multicistronic DNA vaccine induces significant protection against tuberculosis in mice and offers flexibility in the expressed antigen repertoire. Clinical and Vaccine Immunology. 2009 Oct;16(10):1467–75.
66. Whelan KT, Pathan AA, Sander CR, Fletcher HA, Poulton I, Alder NC, et al. Safety and immunogenicity of boosting BCG vaccinated subjects with BCG: Comparison with boosting with a new TB vaccine, MVA85A. PLoS One. 2009 Jun 16;4(6).
67. Smaill F, Jeyanathan M, Smieja M, Medina MF, Thanthrige-Don N, Zganiacz A, et al. A Human Type 5 Adenovirus-Based Tuberculosis Vaccine Induces Robust T Cell Responses in Humans Despite Preexisting Anti-Adenovirus Immunity [Internet]. 2013. Available from: www.ScienceTranslationalMedicine.org
Received: 2 January 2023/ Accepted: 130 March 2023 / Published:15 June 2023
Citation: Zuniga Sanchez R, Sanchez Ramos O , Camacho F.The problem associated with tuberculosis in cattle and how this is being addressed. Revis Bionatura 2023;8 (2) 9. http://dx.doi.org/10.21931/RB/2023.08.02.9