2023.08.03.57
Files > Volume 8 > Vol 8 No 3 2023

Implications of Particle Size in the Extraction of Cellulose from the Calamagrostis Intermedia Species
1 Recursos Naturales Renovables. Facultad de Recursos Naturales. Escuela Superior Politécnica de Chimborazo (ESPOCH); [email protected] .
2 Recursos Naturales Renovables. Facultad de Recursos Naturales. Escuela Superior Politécnica de Chimborazo (ESPOCH); [email protected].
3 Recursos Naturales Renovables. Facultad de Recursos Naturales. Escuela Superior Politécnica de Chimborazo (ESPOCH); [email protected].
* Correspondence: Tel: 0992862598, E-mail: [email protected] (D.R Manzano)
Available from: http://dx.doi.org/10.21931/RB/2023.08.03.57
ABSTRACT
This research aimed to elucidate the implications of particle size on cellulose extraction from the species Calamagrostis intermedia, endemic to Ecuador. Within the versatile cellulose extraction and trade industry, using timber sources results in a relentless environmental impact, mainly when no resource rationing occurs during the washing or bleaching phases. The investigation incorporated a sample pretreatment encompassing a drying, grinding, and sieving study, followed by an alkaline extraction. The study found that particle size significantly affected the quantity of fiber and cellulose pulp extracted from samples previously dried at 65°C with particle sizes of 300 and 106 μm. Characterization tests revealed variations in the color and texture of the extracted cellulose, attributable to an ineffective delignification stage within the fiber bleaching process. Variations in molecular weight corresponding to 126.32 x 103 g/mol were identified among samples, and FTIR analysis presented a syringyl ring and lignin stretching. Optical microscopy exposed the absence of well-defined regions in the fibers and a readily observable crystalline network. In conclusion, particle size critically influences cellulose extraction from Calamagrostis intermedia, impacting the yield and properties of the resultant product, indicating that further optimization of the extraction and delignification process is required to enhance the quality of the extracted cellulose.
Keywords: Calamagrostis intermedia; Particle Size; Cellulose Extraction
INTRODUCTION
Cellulose is a biopolymer comprising hundreds or thousands of carbon, hydrogen, and oxygen atoms 1. It primarily constitutes the cell walls of the plant kingdom, functioning as a structural scaffold that permits plant rigidity 2. The ubiquity of cellulose makes it the most abundant component of the earth's biomass, although its proportion varies with plant species, maturity, and type 3. With the progression of plant growth and cell formation, the polymerization of cellulose naturally occurs through the addition of glucose molecules and subsequent water molecule expulsion. Composed of long chains of beta glucose molecules, cellulose polysaccharides are bound together by glycosidic bonds, with the glucose subunit orientation allowing for the protrusion of OH groups in all directions4,5. These OH groups can form hydrogen bonds with adjacent chains, establishing a three-dimensional network, which is not observed in starch molecules 6,7.
Cellulose exhibits a versatile spectrum of utility across various industries, ranging from animal feed and paper manufacturing to fibers, clothing, and even cosmetic and pharmaceutical sectors 8. Particularly in the pharmaceutical sector, cellulose and its semi-synthetic derivatives, such as cellulose ethers and esters, possess unique physicochemical and mechanical properties, making them valuable for myriad applications 9. They are critical in formulating various pharmaceutical items, such as fillers, taste masking agents, and adhesive agents in transdermal patches, among many other functions 10. The increasing popularity of these polymers within the pharmaceutical industry can be attributed to the continual development of novel derivatives and applications 11. Notably, cellulose ethers, a subset of cellulose derivatives, are commonly employed in bioadhesives and used across various formulations, such as oral, ocular, vaginal, nasal, and transdermal 12, 13.
Beyond its industrial and pharmacological utility, cellulose is the principal raw material in manufacturing paper, cellophane, celluloid, and rayon. It also features prominently in various household items, including plastics, films, synthetic sponges, and as the chief source of dietary fiber. Cellulose is essential in ethanol production through fermentation, marking it as a vital renewable resource 14,15. Hence, cellulose's commercial significance and widespread application cannot be overstated16.
Nevertheless, cellulose extraction from traditional wood sources poses substantial environmental challenges. Wood extraction for cellulose production contributes to the degradation of water resources due to the large amounts of water required for the process and the generation of liquid industrial waste 17,18. This pollution of freshwater bodies is a global issue that extends beyond local environments 19,20.
Given the environmental consequences of cellulose extraction from wood sources, there is a growing urgency to explore non-wood sources. A considerable body of research indicates the potential of non-wood plant species as viable sources of cellulose 22 . However, more in-depth investigations into the extraction process, particularly the influence of particle size in the extraction yield and quality, are needed23 .
The current shortfall in comprehensive understanding highlights the importance of this research, which endeavors to elucidate the role of particle size in the cellulose extraction process from non-wood sources. For this investigation, we selected Calamagrostis intermedia, an endemic Andean straw species widely distributed across Ecuador. Despite its abundance, this particular species currently does not hold a defined industrial use. It grows in vast quantities throughout the Andean region, making it a highly accessible resource. This untapped potential and abundance make it an attractive and sustainable alternative for cellulose production. By turning to this readily available and under-utilized resource, the research contributes to environmental sustainability and can provide a new impetus to local economies. Therefore, this study serves as a crucial exploration into the potential of Calamagrostis intermedia as a non-wood source for cellulose extraction.23 An alkaline extraction method was implemented with a primary focus on investigating the effects of particle size while keeping other extraction variables consistent. The resulting cellulose's characteristics were then correlated with the particle size. This study represents a pivotal step towards environmentally sustainable cellulose production practices, as it delivers a comprehensive analysis of non-wood sources for cellulose extraction and the optimal conditions required for such processes. Consequently, the applied alkaline extraction method aimed to discern the particle size's impacts by managing identical variables during the extraction and correlating the information derived from the characterization tests executed on the extracted cellulose.
MATERIALS AND METHODS
The study was conducted at a laboratory level, considering the peculiarities of industrial extraction methodology. Calamagrostis intermedia, a non-timber species endemic to Ecuador with no defined commercial uses or extractive agriculture, was selected as a source 22. The species is not considered endangered as it is not listed in the Red Book of Endemic Species of Ecuador 23. An experimental design was proposed where the variables of interest are particle size and moisture content related to drying temperature in the samples to be treated for extraction and subsequent characterization.
Raw Material
Samples of the Andean straw species Calamagrostis intermedia were collected at the location -1°28'13.225"S-78°45'1.001" W belonging to the Condor Samana parish within the Guano canton in the Chimborazo Province. It is worth mentioning that during the collection process, the plant roots were not extracted; instead, pruning was carried out at a height of 5 cm from the base, considering samples in which the leaves are at least 90 cm high. After collection, the samples were sent for botanical identification.
Sample Pretreatment
Considering the percentage by weight of water contained in the sample as a reaction intermediate that could disrupt the specificity of reagents in the cellulose extraction process, a drying study was proposed. This was followed by a particle size study that would reveal the influence of this variable on the extraction process. In this manner, 100 g of straw was weighed and placed on each tray. Drying was carried out at temperatures of 65°C and 80°C until a constant weight was achieved, with the weight recorded every hour. The dry straw was crushed and placed in a sieve for 10 minutes at 200 rpm.
Sample Pretreatment Equipment
Electric tray dryer Aio-300
Balance GRAM FS-220
0.5 Hp grinder
Sieve AS 200 RESTCH
Experimental Design
To determine the influence of particle size on cellulose extraction from this non-timber plant species, an experimental design was proposed for the pretreatment of the sample before extraction, simulating laboratory-level methodological conditions. Therefore, the drying temperature was considered a variable because the water molecules bound to the cellulose portions result from the plant's natural polymerization process. This substance, in turn, prevents accurate extraction using a basic solution. Additionally, particle size after drying was considered since grinding depends on the dry portion of the sample 17. In this way, the 22 experimental designs considered that the best analytical result is sought in terms of cellulose and fiber percentage, as well as the physical and chemical characterization of the extracted cellulose, as shown in Table 1.
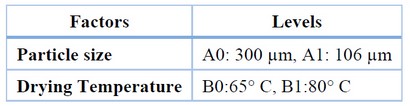
Table 1. Factors and levels of the 2^2 experimental design
Cellulose Extraction
Given the industrial-level peculiarities of the cellulose extraction process, an alkaline extraction was used at the laboratory level. Thus, in a 10% NaOH solution, the Andean straw samples were placed and heated to a temperature of 90° C, maintaining this temperature for 10 minutes. After this time, it was allowed to cool to room temperature. The solid phase was washed with plenty of water until it reached a neutral pH. Then, it was placed in a 4% by-volume sulfuric acid solution. The mixture was boiled for an hour and cooled to room temperature. The solid phase was washed until it reached a neutral pH. The washed sample was placed in a 3.5% volume sodium chlorite solution. The mixture was left to rest for 3 hours and heated for 40 minutes at 95° C. The solid phase was washed until it reached a neutral pH. The sample was placed in a 20% by volume NaOH solution and heated to 50° C on a stir plate at medium speed for 1 hour. The solid phase was washed until it reached a neutral pH. The solid phase was placed in a 0.5% sodium chlorite solution, and the previous step was repeated. Finally, the last wash was carried out until a pH of 7 was obtained, and it was dried for 6 hours at 70° C.
Materials and Reagents for Cellulose Extraction
Electric tray dryer Aio-300
Balance GRAM FS-220
Reverbero PROCTOR SILEX
pH Potentiometer HANNA EDGE
Magnetic Stirrer VEVOR 79-1
Sodium Hydroxide MERCK CAS 1310-73-2
Sulfuric Acid MERCK CAS 7664-93-9
Sodium Chlorite MERCK CAS 7758-19-2
Beakers of 1000, 500, 250 and 100 mL
Watch glass
Spatula
Tweezers
Thermometer
Distilled water
Mesh filter
Timer
Stirring rod
Wash bottle
500mL volumetric flask
10 and 25 mL pipettes
Suction bulb
Characterization of Extracted Cellulose
Molecular Weight Determination
The Spanish Association of Cellulose, Pulp and Paper Manufacturers (ASPAPEL) 24 recommends this widely used characterization test at the industrial and laboratory level. A viscometry analysis was applied using an Ostwald viscometer. A solution of pentahydrate copper sulfate with distilled water, sulfuric acid at 50% volume, and ammonium hydroxide was prepared. To this solution, 0.035 g of extracted cellulose was added, thus preparing solutions of concentration 0.1-0.3-0.5-0.7 g/L for each experiment. Then, 15 mL of cupriethylenediamine standard was placed in the Ostwald viscometer, maintaining a temperature of 25 °C. The previous procedure was repeated with the concentrations of diluted cellulose to evaluate the difference in travel times within the instrument 17,25.
FTIR Analysis
Fourier Transform Infrared Spectroscopy provides information about the molecular structure of a polymer 26, which allows identification according to the obtained spectra. The samples were ground in a mortar until a uniform fine powder was obtained, and they were analyzed within the zone corresponding to 4000 to 600 cm-1. The obtained spectra were reported using the SPECTRAL MANAGER software, and the vibrations in the peaks of each functional group were compared with those reported in samples of cellulose extracted from cotton and wood 27.
Type of Cellulose
The typology of the obtained structural cellulose can be characterized by identifying the position of the hydroxyl group through which the glycosidic bond was generated in the aldose 24. This way, each cellulose sample's solubility was established using 0.1g of cellulose in 10 mL of NaOH at 17.5% and 8% volume.
Optical Microscopy
This analysis is of interest since, in the chemical industry and the manufacturing field, polymers are observed under the microscope when observing the morphology of the crystal, spherulites, fracture plane surfaces, process control, failure analysis, roughness, deformation, quantitative and qualitative analysis of the microstructure and when observing interference patterns 28. Therefore, a direct observation at an amplitude of 40 and 60 X was applied to the extracted cellulose samples, comparing the regions and images with those reported in samples of cellulose extracted from cotton and wood.
Materials and Equipment in the Characterization
Mortar
Spatula
Distilled water
Spatula
Stirring Rod
50mL beaker
Test tubes
50 mL graduated cylinder
Pentahydrate copper sulfate AVALCO
Ammonium hydroxide BIOPACK
Sodium Hydroxide MERCK CAS 1310-73-2
Sulfuric Acid MERCK CAS 7664-93-9
Balance GRAM FS-220
IR Spectrophotometer JASCO FT/IR-4100typeA
Optical Microscope UNICO M280
Ostwald viscometer YUCHENGTECH
Stopwatch
RESULTS
Taxonomic Identification of the Samples
Based on the morphology and taxonomy analyzed in the collected samples and compared with patterns identified in the Alfredo Paredes Herbarium of the Central University of Ecuador, the samples in this study were determined to belong to the Poaceae family, specifically the species Calamagrostis intermedia (J. Presi) Steud. This confirmation establishes the specimen as a non-timber species, endemic to Ecuador and not endangered.
Sample Drying
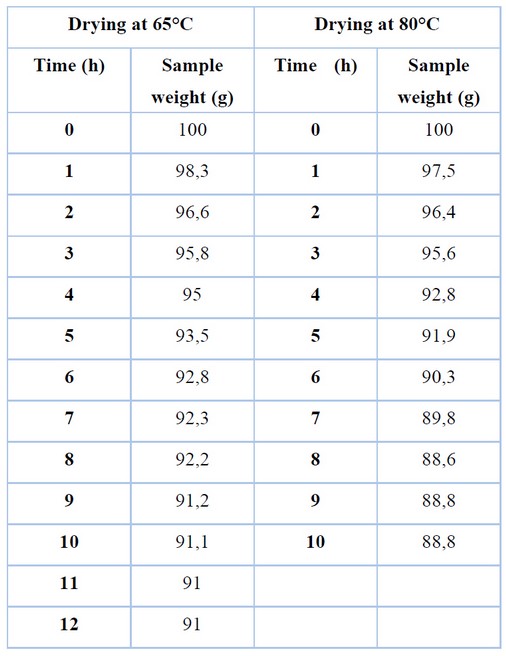
Table 2. Sample Drying
Table 2 illustrates the sample mass recorded every hour during drying at two different temperatures. While the sample at 65°C maintains a consistent mass for 11 hours (thus marking the post-critical point in the drying kinetics), this point is reached at 8 hours when the temperature is set at 80°C. However, 9 and 12.2 g losses are registered at 65° and 80° C respectively. The data suggests that the sample contains approximately 10% water before extraction.
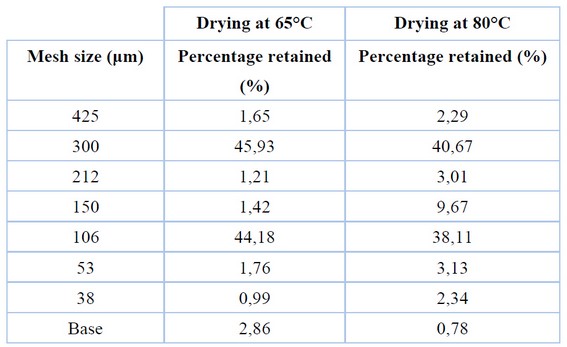
Table 3. Sieving of the Sample
Table 3 presents the results of the sieving study applied after grinding the dried sample. It shows that particle sizes corresponding to the 300 and 106 μm mesh apertures retain the most mass in samples treated at 65 and 80°C.
Experimental Design
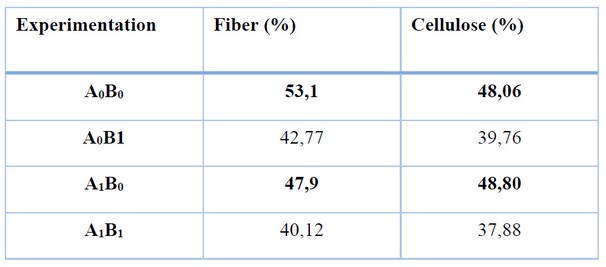
Table 4. Fiber and Cellulose Percentage Recovered in the Conjugation of Experimental Variables
To determine the percentage of cellulose and fiber in each experiment where the experimental design was applied, the following formulas were used:
Cellulose Fiber = X/P x 100 (1)
Where:
X = quantity of fiber (g)
P = quantity of dried straw (g)
Cellulose Yield = C/Z x 100 (2)
Where:
C = quantity of pulp (g)
Z = Cellulose fiber (g)
It is established that the cellulose extraction process presenting the highest mass of fiber and cellulose pulp is the one considering samples previously dried at 65°C and particle sizes of 300 and 106 μm.
Cellulose Extraction
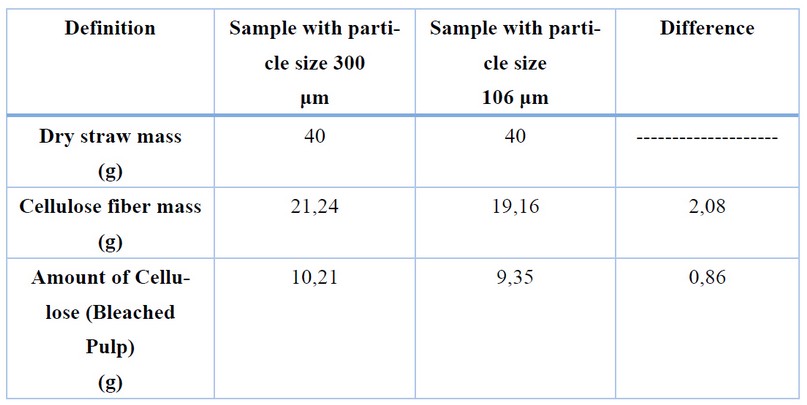
Table 5. Fiber Mass and Extracted Cellulose Pulp in Samples of Definitive Particle Size
Table 5 highlights the difference in the mass quantity of fiber and extracted cellulose pulp in samples of 300 and 106 μm particle size. Although this difference is minimal, Figure 1 demonstrates a significant variance in the color and texture of the extracted cellulose pulp, presumably due to an inefficient delignification process that hinders adequate fiber swelling and poor cellulose release, showcasing the influence of particle size on the extraction.
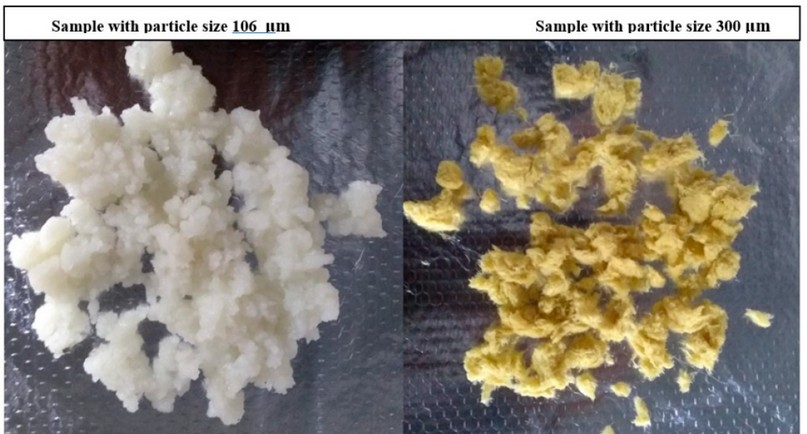
Figure 1. Extracted Cellulose Pulp from Different Particle Sizes.
Cellulose Characterization
Although the cellulose extracted from the 300 μm sample appears yellowish. With the typical structure of cellulose fiber, characterization tests were applied in both cases to determine the influence of particle size and its effect on the structural properties of the extracted cellulose.
Molecular Weight
Figure 2 shows the extrapolation when the concentration tends to zero, considering five experiments to determine the reduced viscosity of the sample. In this way, it is possible to replace the value of this viscosity in the Mark Houwink equation, considering the constants and parameters described in the literature 25. In this way, the molecular weight obtained in the cellulose extracted from the 106 and 300 μm sample size is 292.83 x 103 g/mol and 419.15 x 103 g/mol, respectively. A notable difference of about 126.32 x 103 g/mol is observed in this characterization test. This magnitude of difference is assumed to be related to the amount of lignin present in the fibers of the 300 μm sample. However, both samples are within the optimal standard molecular weight of industrial-grade cellulose.
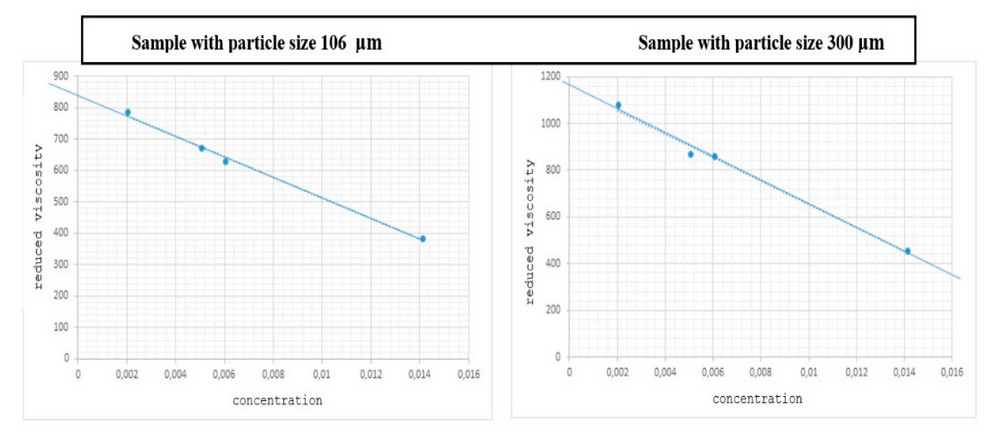
Figure 2. Determination of reduced viscosity in the samples
FTIR Analysis
Figure 3 shows the spectra and vibrations obtained in the samples of cellulose extracted from defined particle sizes. In this way, it is possible to characterize these polymers based on the percentage of transmittance present in each vibration assigned to a specific functional group, thereby providing precise structural information about the type of polymer obtained. Generally, both spectra show very similar patterns in vibrations and transmittance percentages. The observed wave numbers are similar for hydroxyl functional groups, C-H stretching in methyl and methylene groups, non-conjugated ester groups, bending in cellulose and hemicellulose, cellulose and hemicellulose C-O-C, CH and C-O- deformations. However, in the wave numbers corresponding to the syringyl ring and C-O stretching in lignin and xylan, a value of 1268.93 cm-1 is found in the 300 μm sample, while this peak is not evident in the 106 μm sample. Therefore, the inefficiency of the delignification process in this sample is rectified.
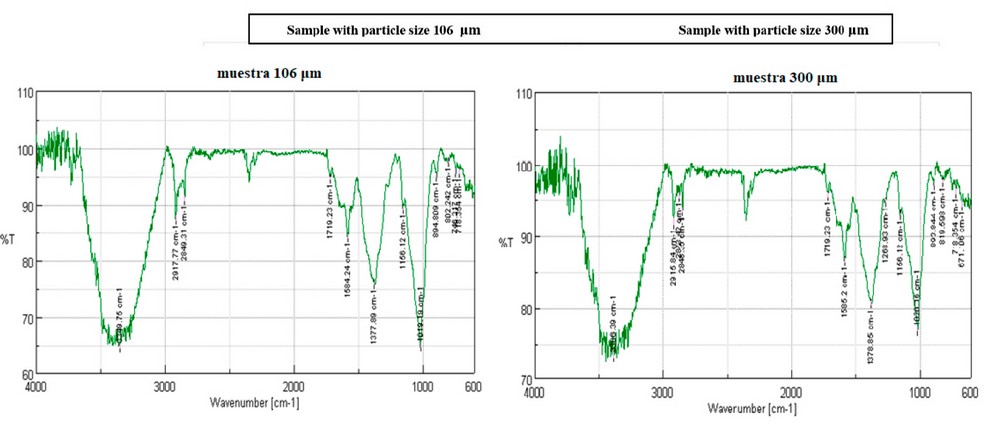
Figure 3. FTIR analysis of cellulose samples extracted from particle sizes of 106 and 300 μm
Type of Cellulose
About the solubility of the samples in 17.5% and 8% sodium hydroxide solutions, it was determined that the cellulose present in the plant species corresponds to the beta type as it is soluble in a concentration of 17.5% volume of sodium hydroxide.
Optical Microscopy
Upon applying this characterization analysis, very intertwined fibers are observed in both samples. However, in the cellulose sample extracted from a particle size of 106 μm, the fiber contours are even and present clear crystallinity, which is expected in high-purity polymers. Conversely, the fibers are noticeably thicker in the cellulose extracted from the 300 μm particle size and no defined contours with crystallinity are observed.
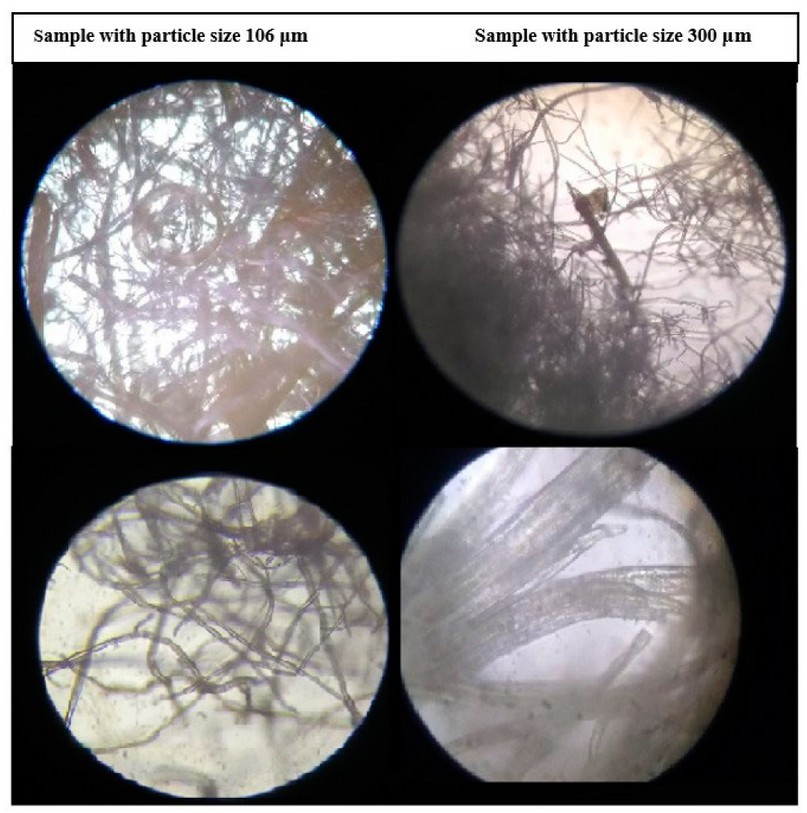
Figure 4. Optical microscopy of cellulose samples extracted from particle sizes of 106 and 300 μm.
DISCUSSION
The results derived from this investigation reveal several intriguing aspects concerning the extraction and characterization of cellulose from different particle sizes. Firstly, the molecular weight results suggest that the lignin content within the fibers may considerably impact the final molecular weight. There was a notable difference of approximately 126.32 x 103 g/mol between the cellulose extracted from the 106 μm and 300 μm samples. This variance could be attributed to the higher lignin content in the 300 μm sample, which would indeed increase the overall molecular weight29. However, both samples still fall within the optimal standard molecular weight range for industrial cellulose, suggesting that the particle size does not significantly influence the applicability of the extracted cellulose.
Our FTIR analysis revealed similar spectra and vibrations for both extracted cellulose samples, indicating that the polymer structure remains unchanged despite the particle size difference. Both spectra exhibited functional groups related to cellulose, such as hydroxyl, C-H stretching in methyl and methylene groups, unconjugated ester groups, cellulose and hemicellulose C-O-C, CH deformation, and C-O- stretching. Yet, the 300 μm sample peaked at 1268.93 cm-1, indicative of syringyl ring and C-O stretching in lignin and xylan. The absence of this peak in the 106 μm sample implies a more efficient delignification process.
The cellulose type was determined to be beta cellulose based on its solubility in 17.5% sodium hydroxide. This type of cellulose is a standard variety found in many plant species, and its identification aligns with prior research26.
Optical microscopy presented a detailed visual of the fiber structure. The cellulose extracted from the 106 μm particles exhibited even contours and a clear crystallinity, characteristic of highly pure polymers12. However, the cellulose from the 300 μm particles was noticeably thicker. It lacked well-defined contours and crystallinity, possibly due to the less efficient delignification process and higher lignin content.
Together, these results offer valuable insights into the role of particle size in cellulose extraction and characterization. However, further research is needed to comprehensively understand the intricate interplay between particle size, lignin content, and cellulose properties. Additionally, developing and optimizing more efficient delignification processes could improve cellulose purity and molecular weight consistency, thereby widening its applicability in industrial processes 30.
CONCLUSIONS
The core of this study revolves around a non-timber plant species, classified based on the patterns identified at the Alfredo Paredes Herbarium of the Central University of Ecuador. Specifically, the species in question belongs to the Poaceae family and is taxonomically identified as Calamagrostis intermedia (J. Presi) Steud. This species is endemic to Ecuador and is currently not at risk.
The investigation analyzed the influence of particle size on the cellulose extraction process and found that the highest fiber and cellulose pulp mass was achieved with particle sizes of 300 and 106 μm, pre-dried at 65°C. Characterization tests revealed notable variations in the color and texture of the extracted cellulose. Cellulose derived from the 300 μm particle size was yellowish due to incomplete delignification during bleaching.
The characterization tests further substantiated these findings, with both samples meeting industrial cellulose's quantifiable and qualitative parameters. Nonetheless, differences were observed in molecular weight, with a divergence of 126.32 x 103 g/mol between the samples. The FTIR analysis identified a wavenumber of 1268.93 cm-1, corresponding to the syringyl ring and C-O stretching in lignin and xylan. Optical microscopy demonstrated that cellulose extracted from the 300 μm particle size lacked well-defined regions within the fibers and a discernable crystalline network.
This study posits that particle size significantly influences the delignification, bleaching of pulp, and the swelling process of fibers, facilitating the release of cellulose within plant cell walls. This effect is closely tied to the concept that an increased surface area heightens the efficiency of the chemical reaction. When particle size permits a larger contact surface between the reactant and reagent, the reaction yield improves. This principle is decisive in determining the properties and influences on reaction kinetics. Hence, under the conditions of this study, a larger contact surface area leads to a more efficient bleaching reaction.
It was ascertained that the plant species contains beta-type cellulose, which carries significant implications for its potential uses and processing techniques. These results validate the importance of particle size in cellulose extraction and suggest the potential of underutilized plant species like Calamagrostis intermedia as viable alternatives for sustainable cellulose production.
Given the successful extraction and characterization of cellulose from Calamagrostis intermedia, the authors suggest that future investigations could expand this approach to other species within the Poaceae family or even beyond to diversify non-timber sources of cellulose further. Species like Miscanthus giganteus, a high-yield perennial grass, or even Bamboo species, which proliferate and are known for their high cellulose content, could be potential candidates for future research. Other native grasses that are abundant and underutilized in different regions around the world, such as switchgrass (Panicum virgatum) or vetiver (Chrysopogon zizanioides) in tropical regions, may also prove to be promising sources of cellulose.
The findings of this study pave the way toward a more sustainable cellulose industry, moving away from the current environmentally detrimental practices. The authors propose that future research consider other taxonomically similar species, expanding the scope of non-timber alternatives for cellulose extraction. Implementing such findings into practice could significantly lessen the environmental impact and create a path toward a more sustainable cellulose industry..
Patents
Does not apply.
Acknowledgments: Foremost, the group of authors involved in this study would like to express their sincere gratitude to the Alfredo Paredes Herbarium of the Central University of Ecuador for their generous assistance with the botanical identification of the plant species examined. Similarly, our most profound appreciation goes to the Escuela Superior Politécnica de Chimborazo, particularly the Faculty of Natural Resources authorities, for providing the academic support necessary for this research.
Conflicts of Interest: No conflicts of interest are reported.
REFERENCES
1. Radotić K, Mićić M. Methods for Extraction and Purification of Lignin and Cellulose from Plant Tissues. 2018;365–76.
2. de Dios Naranjo C, Alamilla-Beltrán L, Gutiérrez-Lopez GF, Terres-Rojas E, Solorza-Feria J, Romero-Vargas S, et al. Aislamiento y caracterización de celulosas obtenidas de fibras de Agave salmiana aplicando dos métodos de extracción ácido-alcali*. Rev Mex De Cienc Agric. 2016;7:31–43.
3. Tudoroiu EE, Dinu-Pîrvu CE, Kaya MGA, Popa L, Anuța V, Prisada RM, et al. An Overview of Cellulose Derivatives-Based Dressings for Wound-Healing Management. Pharmaceuticals [Internet]. 2021 dic 1 [citado 2022 sep 1];14(12):1215. Available from: /pmc/articles/PMC8706040/
4. Manzano Vela DR, Novillo Logroño FA. Preparation and Characterization of Cellulose Acetate and Cellulose Nitrate Prepared from Cellulose Extracted from Calamagrostis intermedia. Asian Journal of Chemistry [Internet]. 2022 jul 18 [citado 2022 sep 1];34(8):2099–104. Available from: https://asianjournalofchemistry.co.in/user/journal/viewarticle.aspx?ArticleID=34_8_22
5. Hon DNS, Shiraishi N. Wood and Cellulosic Chemistry, Revised, and Expanded. Wood and Cellulosic Chemistry, Revised, and Expanded. 2015.
6. Vega-Baudrit J, Sibaja M, Nikolaeva S, Rivera A. Síntesis Y Caracterización De Celulosa Amorfa. Revista Sociedad Química de Perú. 2014;80(1):45–50.
7. Romero P, Marfisi S, Oliveiros P, Rojas B, Peña G. Obtención De Celulosa Microcristalina a Partir De. 2014;15(6):286–300.
8. Chen D, Gao A, Cen K, Zhang J, Cao X, Ma Z. Investigation of biomass torrefaction based on three major components: Hemicellulose, cellulose, and lignin. Energy Convers Manag [Internet]. 2018;169(April):228–37. Available from: https://doi.org/10.1016/j.enconman.2018.05.063
9. Henao L, Rojas I, Giraldo G. Cuantificación de celulosa proveniente de residuos de la industria del mueble. Ingenieria de Recursos Naturales y del Ambiente. 2009;8:23–8.
10. Wu JH, He CY. Advances in Cellulose-Based Sorbents for Extraction of Pollutants in Environmental Samples [Internet]. Vol. 82, Chromatographia. Friedr. Vieweg und Sohn Verlags GmbH; 2019 [citado 2021 mar 7]. p. 1151–69. Available from: https://link.springer.com/article/10.1007/s10337-019-03708-x
11. Sanchez S, Garcia Y, Carlos L, Falconi R, Veleva L. METODOS PARA EXTRAER CELULOSA DE LA PAJA DE CANA DE AZUCAR (Saccharum spp.). AGROProductividad [Internet]. 2017 nov 1 [citado 2020 ago 26];10(11):54–60. Available from: https://go.gale.com/ps/i.do?p=IFME&sw=w&issn=&v=2.1&it=r&id=GALE%7CA530914347&sid=googleScholar&linkaccess=fulltext
12. Orelma H, Hokkanen A, Leppänen I, Kammiovirta K, Kapulainen M, Harlin A. Optical cellulose fiber made from regenerated cellulose and cellulose acetate for water sensor applications. Cellulose [Internet]. 2020 feb 1 [citado 2021 mar 7];27(3):1543–53. Available from: https://doi.org/10.1007/s10570-019-02882-3
13. Golubev AE, Kuvshinova SA, Burmistrov VA, Koifman OI. Modern Advances in the Preparation and Modification of Cellulose Nitrates. Russ J Gen Chem [Internet]. 2018 feb 1 [citado 2021 mar 7];88(2):368–81. Available from: https://link.springer.com/article/10.1134/S1070363218020305
14. Shokri J, Adibkia K. Application of Cellulose and Cellulose Derivatives in Pharmaceutical Industries. Cellulose - Medical, Pharmaceutical and Electronic Applications [Internet]. 2013 ago 29 [citado 2022 sep 1]; Available from: undefined/state.item.id
15. Agwuncha SC, Anusionwu CG, Owonubi SJ, Rotimi Sadiku E, Busuguma UA, David Ibrahim I. Extraction of cellulose nanofibers and their eco/friendly polymer composites. En: Sustainable Polymer Composites and Nanocomposites [Internet]. Springer International Publishing; 2019 [citado 2021 Mar 7]. p. 37–64. Available from: https://link.springer.com/chapter/10.1007/978-3-030-05399-4_2
16. Singh A, Ranawat B, Meena R. Extraction and characterization of cellulose from halophytes: next-generation cellulose fiber source. SN Appl Sci [Internet]. 2019 nov 1 [citado 2021 mar 7];1(11):1–10. Available from: https://doi.org/10.1007/s42452-019-1160-6
17. Herman M. Encyclopedia of Polymer Science and Technology. 3a ed. New York; 2012. 226 p.
18. Huda E, Rahmi, Khairan. Preparation and characterization of cellulose acetate from cotton. IOP Conf Ser Earth Environ Sci. 2019;364(1).
19. Vazquez A, Foresti ML, Moran JI, Cyras VP. Extraction and production of cellulose nanofibers. En: Handbook of Polymer Nanocomposites Processing, Performance and Application: Volume C: Polymer Nanocomposites of Cellulose Nanoparticles [Internet]. Springer Berlin Heidelberg; 2017 [citado 2021 mar 7]. p. 81–118. Available from: https://link.springer.com/chapter/10.1007/978-3-642-45232-1_57
20. Amaral HR, Cipriano DF, Santos MS, Schettino MA, Ferreti JVT, Meirelles CS, et al. Production of high-purity cellulose, cellulose acetate and cellulose-silica composite from babassu coconut shells. Carbohydr Polym. 2019 abr 15;210:127–34.
21. Agarwal S. Biodegradable Polymers: Present Opportunities and Challenges in Providing a Microplastic‐Free Environment. Macromol Chem Phys [Internet]. 2020 mar 26 [citado 2021 jun 27];221(6):2000017. Available from: https://onlinelibrary.wiley.com/doi/abs/10.1002/macp.202000017
22. Emenike EC, Iwuozor KO, Saliu OD, Ramontja J, Adeniyi AG. Advances in the extraction, classification, modification, emerging and advanced applications of crystalline cellulose: A review. Carbohydrate Polymer Technologies and Applications. 2023 Dec;6:100337.
23. Hagiopol C. Trees: a sustainable source of lignin and tannin. Natural Polyphenols from Wood [Internet]. 2021 Jan 1 [cited 2023 Aug 6];7–32. Available from: https://linkinghub.elsevier.com/retrieve/pii/B9780128222058000037
24. Romoleroux K, Cárate-Tandalla D, Navarrete R. Calamagrostis intermedia En: Plantas vasculares de los bosques de Polylepis en los páramos de Oyacachi. Version 2019. [Internet]. 2019. Available from: https://bioweb.bio/floraweb/polylepis/FichaEspecie/Calamagrostis intermedia
25. Ministerio de Ambiente del Ecuador., Flora Web Ecuador, Pontifícia Universidad Católica del Ecuador. Libro Rojo de Plantas Endémicas del Ecuador [Internet]. Herbario QCA. 2019 [citado 2021 abr 28]. Available from: https://bioweb.bio/floraweb/librorojo/ListaEspeciesPorFamilia/500363
26. TAPPI. Alpha- , beta- and gamma-cellulose in pulp. Test Methods T 203 cm-99. Atlanta: Technical Association of the Pulp and Paper Industry. 1999.
27. Wypych G. Handbook of Polymers [Internet]. 2a ed. ChemTec, editor. Toronto: ChemTec; 2016. 705 p. Available from: http://www.manaepd.com/images/handbook of polymer.pdf
28. Fuller ME, Andaya C, McClay K. Evaluation of ATR-FTIR for analysis of bacterial cellulose impurities. J Microbiol Methods. 2018 ene 1;144:145–51.
29. Bao Y, Luo Z, Cui S. Environment-dependent single-chain mechanics of synthetic polymers and biomacromolecules by atomic force microscopy-based single-molecule force spectroscopy and the implications for advanced polymer materials [Internet]. Vol. 49, Chemical Society Reviews. Royal Society of Chemistry; 2020 [citado 2021 Jun 27]. p. 2799–827. Available from: https://pubs.rsc.org/en/content/articlehtml/2020/cs/c9cs00855a
30. Gentekos DT, Sifri RJ, Fors BP. Controlling polymer properties through the shape of the molecular-weight distribution. Nature Reviews Materials [Internet]. 2019 dic 1 [citado 2021 mar 8];4(12):761–74. Available from: https://www.nature.com/articles/s41578-019-0138-8
Received: 25 June 2023/ Accepted: 26 August 2023 / Published:15 September 2023
Citation: Manzano Vela D R , Zabala Vizuete R F, Flores Mancheno A .Implications of Particle Size in the Extraction of Cellulose from the Calamagrostis Intermedia Species, Revis Bionatura 2023;8 (3) 57. http://dx.doi.org/10.21931/RB/2023.08.03.57