2022.07.03.58
Files > Volume 7 > Vol 7 No 3 2022

Evaluation of the concentration of heavy metals in vegetables from Ecuador
1 Faculty of Natural Sciences, University of Guayaquil, Av. Raúl Gómez Lince s/n y Av. Juan Tanca Marengo, Guayaquil, Ecuador
2 Center of Studies for Sustainable Development, University ECOTEC, Km 13.5 vía Samborondón, Guayaquil, Ecuador.
3 Natural Resources Research Institute, Faculty of Natural Sciences, University of Guayaquil, Av. Raúl Gómez Lince s/n y Av. Juan Tanca Marengo, Guayaquil, Ecuador.
Corresponding author: [email protected]
Available from: http://dx.doi.org/10.21931/RB/2022.07.03.58
ABSTRACT
Heavy metals are toxic elements that have contaminated agricultural waters and soils due to their use in anthropic activities, generating bioaccumulation in food and biomagnification in the food chain. In this research, information was collected on the presence of heavy metals in different foods of plant origin in Ecuador, the first link in the trophic chain, to establish a baseline in the field of food toxicology. Information was extracted from the central databases, scientific journals and libraries' digital repositories, analyzing data on Cd, Hg, Pb and the metalloid As in 15 plants. According to the Mercosur standard, 14 exceeded the maximum permissible limit (LMP) of As, distinguishing the avocado (19.76 ± 1.36 mg/kg As). According to the Codex Alimentarius, 31% of the vegetables exceeded the LMP in terms of Cd; carrots (9.71 ± 8.66 mg/kg Cd) were the most contaminated. Mercury (Hg) was studied only in potatoes (0.04 mg/kg Hg), which was found to exceed the standard four times, while Pb surpassed the limits in 33% of the samples analyzed, sugarcane the vegetable with the highest concentrations of 4.32 mg/kg Pb. In conclusion, multi-metal contamination was evidenced in some vegetables, representing a risk to consumers' health and food safety.
Keywords: bioremediation, contamination, food safety, heavy metals, toxicity
INTRODUCTION
Heavy metals are hazardous pollutants due to their toxicity, persistence in the environment, ability to accumulate in organisms, and biomagnification in the food chain 1. Industrialization and agrochemical use have generated contamination by heavy metals in agricultural soils. These metals are easily absorbed by the roots and transported to the edible parts of the plant, ultimately entering the food chain 2. Consequently, plants accumulate these elements, generating several diseases in consumers3.
Contamination in food can originate naturally, due to environmental contamination, as a consequence of bad agricultural practices or the mishandling of food on the way from field to table. Therefore, food safety assessment is challenging given the diversity of foods and agricultural products 3.
Among the most toxic heavy metals are Cadmium (Cd), lead (Pb) and mercury (Hg), in addition to the metalloid arsenic (As). The latter has generated public health problems in the United States, Mexico, Chile, Argentina, Bangladesh, India, China, Taiwan and Thailand, where the cases of lung, bladder, and skin cancer associated with the consumption of water contaminated with As have increased 4,5. Contamination by this metal has been reported in rice in Australia 6, Bangladesh 7and Brazil 8 as has also been detected in wheat, corn and potatoes 9,10.
As causes some serious health effects such as anemia, melanosis (hyperpigmentation or dark spots and hypopigmentation or white spots), hyperkeratosis (hardening of the skin), restrictive lung disease, peripheral vascular disease (black foot disease), gangrene, diabetes mellitus, hypertension, ischemic heart disease, and cancer 11,12.
On the other hand, chronic exposure to Cd mainly affects the kidneys, causing cardiac nephropathy 13,14, high blood pressure, diabetes, cardiovascular effects 15,16, lung and prostate cancer 13,17, endocrine disruptions related to breast and endometrial cancer 17, teratogenesis and mutagenesis 18–20. Additionally, Cd can damage calcium (Ca) metabolism, resulting in a Ca deficiency that can cause bone fractures and cartilage damage 21.
A classic example of excessive consumption of Cd through contaminated food is the "Itai-Itai" disease (osteomalacia) described in Japan because of the consumption of contaminated rice. Other foods where contamination with Cd has been described are lettuce, peanuts, spinach and soybeans because these plants can accumulate heavy metal in high concentrations, and their hyper-accumulative capacity has been demonstrated 22. Likewise, high levels of Cd in potatoes have been found in Brazil 10.
Another essential metal is Hg, which can come from natural sources, but is mainly associated with anthropogenic activities, particularly the extraction and use of fossil fuels and gold 23. Mercury has several presentations; inorganic Hg is the form in which it is discharged into aquatic systems, and in the upper layers of aquatic sediments and water, bacteria transform inorganic Hg into methylmercury 24.
Methylmercury is the most toxic form, highly bio-accumulative in organisms and biomagnifies in the food chain 25. Hg, in its form of methylmercury, produces acrodynia or pink disease. The Environmental Protection Agency (EPA) has declared that methylmercury is highly carcinogenic, and its exposure can alter brain functions and cause shyness, memory problems, tremors, irritability and changes in vision or hearing. Excess Hg consumption leads to Minamata disease, as reported in Japan, which is characterized by symptoms of memory loss, fatigue, cortical blindness, and poor motor control 26. Numerous authors have also described the contamination of foods of plant origin, where spinach, mint, onion and carrot are mentioned 27–29.
Regarding Pb, since ancient times, poisoning by this metal has been known to generate diseases such as lead poisoning (or plumbism) caused by storing food in Pb containers 30,31. The main signs of Pb poisoning are predominantly related to the gastrointestinal tract and the central nervous system 32-33. Its chronic exposure can cause congenital disabilities, muscle weakness, weight loss, kidney damage, allergies, and brain damage, including mental retardation, autism, hyperactivity, psychosis, dyslexia, and paralysis, and can even result in death 34. In vegetables, Pb contamination has been described in corn, potatoes, paprika and spinach 35,36.
In Ecuador, 8% of the gross domestic product depends on agriculture 37. There are 8.81 million hectares with agricultural uses 38 exposed to fertilizers and pesticides that may contain heavy metals that contaminate the soil and enter the food and, consequently, generate diseases in the population.
In Ecuador, there is evidence of contamination by heavy metals in children and pregnant women. In this sense, high lead levels were found in breast milk (3.73 µg / L, max. 28 µg / dL) and blood (7.8 µg / dL, max. 21 µg / dL) of lactating women from the Ecuadorians Andes 39, and in the city of Guayaquil the presence of Pb in the blood (0.7 µg / L, max. 2.2 µg / dl) was evidenced in people not exposed to this heavy metal at work 40. On the other hand, there is occupational exposure to lead in battery factory workers in the city of Guayaquil (3.80- 38.30 μg/dL) 40 and in sites of widespread production of Pb glazed tiles and other ceramics in the south of Quito41. In children from the population of La Victoria and El Tejar, values of 6.6-119.1 µg / dL were found to be associated with the consumption of water, food, and dust contaminated with lead as a result of glazing of ceramics and were reflected in the health of children as impaired visual pursuit, a deficit in cognitive/perceptual abilities in math calculation skills, neurological deficits in fine motor, perceptual, and non-verbal reasoning skills 41, and in adults, sensory-neural hearing loss in men, which may be attributable to occupational noise exposure in combination with Pb intoxication 42. However, these investigations have not considered the intake of food contaminated with heavy metals.
In another study, high concentrations of Pb, Cd, and As 43 were found in the blood of mothers and children from the Ecuadorian Andes and associated with factors such as residence in a dirt-floor home, living near a dirt-paved or cobblestone street, or in local neighborhoods where scrap metal smelters and gas depositories; as well as other anthropogenic and geogenic sources43. These authors also mentioned the possible intake of food contaminated with heavy metals as a potential source of contamination43.
According to Globoscan44, the most frequent types of cancer in Ecuador are breast, prostate, colorectal, and stomach cancer. In 2020, there were 29,273 new cancer cases with a mortality of 15,123 people 44. The incidence rates of gastric cancer are expected to increase in Ecuador through 2030 45. Another study showed that there had been an increase in the incidence and mortality rates of breast cancer in Ecuador in the last five years46. We wonder if this increase in cancer cases is due to the intake of heavy metals, the lack of food monitoring, and policies that require compliance with standards in food for national consumption described in the Codex Alimentarius. As a food exporting country, Ecuador has strict controls on export products, regulated with the standards of the European Union and the Codex Alimentarius; however, those rules are not applied to products for the national consumption market.
In light of this evidence, in this research, we decided to study the sources of contamination, targeting the food supplies through the compilation of information on the presence and the amounts of heavy metals in different foods of plant origin, the first link in the trophic chain, comparing to the maximum permissible levels established by the national and international standards to determine a baseline in the field of food toxicology in Ecuador
METHODS
The Pubmed, Scopus and Web of Science databases were extensively searched for previous reports. Finding little information, the keywords (heavy metals, Cadmium, lead, mercury, arsenic, vegetables, crop plants) were searched in Google Scholar and in the Digital Repositories of Libraries, focusing on Ecuador. Subsequently, the investigations carried out between the years 2010 to 2021 that met the inclusion criteria of being either scientific articles, theses or reports were selected, taking into account those whose laboratory analyses had reference material or had been carried out by entities with the parameters of heavy metals accredited under the ISO 17025 standard, to ensure the validity of the information (Table 1).
DATA ANALYSIS
A descriptive analysis was carried out for each variable. The means, standard deviations, maximums, and minimums were calculated by location and food for each heavy metal. Dendrograms were elaborated to analyze the grouping of vegetables concerning the metal concentrations. All analysis and graphs were performed using R Studio version 4.0.2 programs. To prepare maps, the location coordinates were homogenized to the projected coordinate system UTM WGS 84 using the Google Earth program and plotted on maps for identifying critical points of contamination by heavy metals using the ArcGIS Program. The results were compared with international standards of maximum permissible limits (MPL) in food, including among them, the Codex Alimentarius of the World Health Organization (WHO and FAO), the standard of the European Union (2019) and the MERCOSUR regulation (2011).
Arsenic (As) detected in products of plant origin
The collected studies showed As a presence in 15 plants, of which 14 exceeded the maximum permissible limits (Table 2). Table 2 shows that the highest concentration occurred in the Persea americana fruit (avocado) with an average of 19.76 mg/kg As recorded in the town of Izamba, Ambato canton 47. Avocado exceeded 66.9 times the maximum permissible limit (MPL) established by MERCOSUR (0.3 mg/kg As) 48. This could be related to its long-life cycle; since being a perennial plant, it can accumulate large amounts of pollutants from the cultivation soil, especially in soils of volcanic origin, irrigation water and the type of agrochemicals used for its production.
Plant species beetroot (Beta vulgaris) and cabbage (Brassica oleracea) also presented high concentrations of As (5.75 ± 3.53 mg/kg; 4.89 ± 1.62 mg/kg, respectively). The food with the lowest As concentration was potato (Solanum tuberosum) with 0.80 mg/kg As determined in two farms in the Mejía canton 49, Pichincha province.
Figure 1A shows an association dendrogram where several groups are distinguished: the first, where the Persea americana species is found, showing the highest accumulation of As; another group formed by Lactuca sativa, Oryza sativa and Solanum tuberosum, which represents the species with the lowest accumulation of As and the third group made up of the species with intermediate concentrations: Beta vulgaris, Brassica oleracea var. botrytis, Apium graveolens, Brassica rapa pekinensis, Allium fistulosum, Rubus ulmifolius, Brassica oleracea var. capitata, Fragaria x ananassa, Brassica oleracea var. capitata, Solanum lycopersicum and Spinacia oleracea.
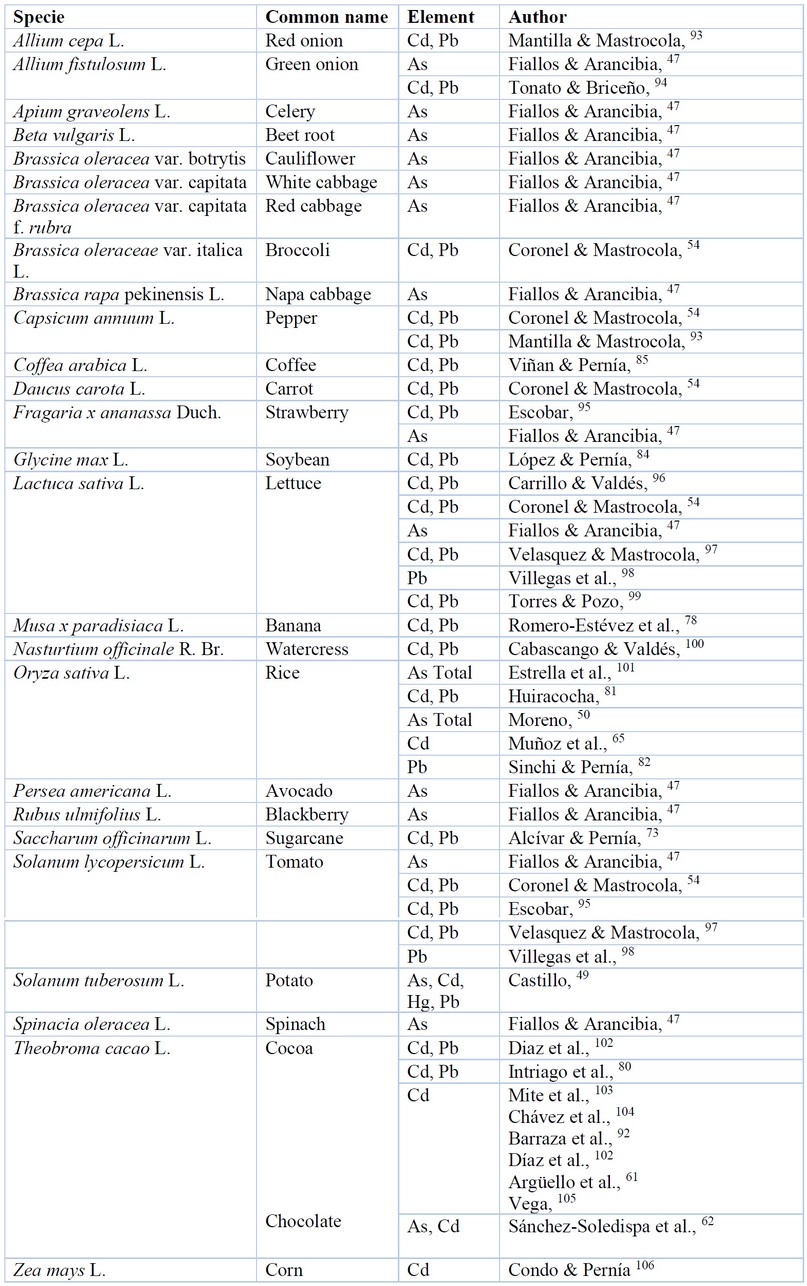
Table 1. Summary of the food of plant origin analyzed in Ecuador
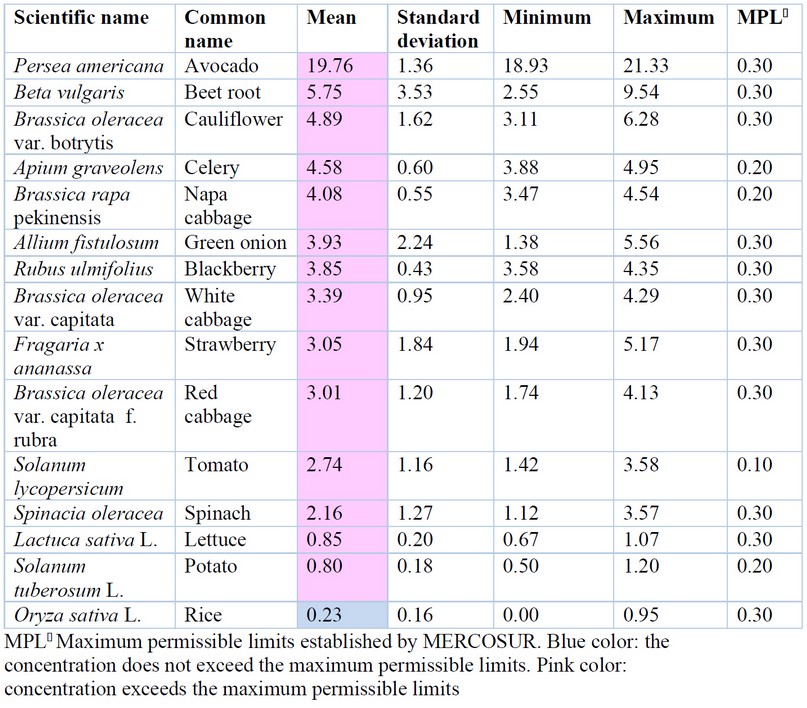
Table 2. Summary of arsenic (As) concentration in Ecuadorian vegetables and its comparison with the maximum permissible limits (MPL).
All the As in plant foods averages exceeded the MPL according to the MERCOSUR standard 48, except for rice (Table 1). However, Oryza sativa presented maximum values above the LMP in the provinces of El Oro, Guayas and Los Ríos, with the highest concentration of 0.95 mg/kg As being observed in the Vinces canton 50.
These high concentrations of As in food could be due to the influence of volcanoes, one of the leading natural sources of As in Latin America 51. In addition, it must be taken into account that the foods analyzed were found in the area of influence of the Tungurahua volcano (except for rice), which presented volcanic activity on the sampling dates of study 47; therefore, the accumulation of As could be due to deposition of volcanic ash.
Likewise, volcanic rocks are a natural contribution of As in aquifers, presenting values between 100 to 794 μg / L As in geothermal water bodies. These water bodies can be contributors to surface waters; however, anthropogenic sources play a significant role in the distribution of As in these tributaries 52, hence the importance of carrying out As analysis in irrigation waters. It is essential to mention that the national regulations of Ecuador do not contemplate the arsenic content for agricultural products; therefore, the reported As content was compared with the international regulations. This is an essential factor to consider due to the strong influence of this metalloid in volcanic areas of Ecuador and the high levels of As found in the present work. In addition, the speciation of As should be considered since inorganic arsenic is more toxic than organic, and most of the analyzed studies measured total As. Likewise, digestibility tests should be carried out to evaluate the risk for the Ecuadorian population due to the consumption of these vegetables.
Cadmium (Cd) presence in products of plant origin
Regarding Cd, Table 3 shows that of the 17 plant species studied, 5 exceeded the MPL according to the Codex Alimentarius 53. Carrot (Daucus carota) presented the highest concentration with 9.71 mg/kg, and it is important to pinpoint that it was obtained in organic fairs in Quito 54. This represented 97.06 times more Cd than the MPL for roots, according to Codex Alimentarius (0.1 mg/kg). This value is extremely high compared to that obtained in China and Bangladesh (0.023 mg/kg Cd) 55-56. The high content of Cd in carrots maybe because it is a storage root, and it has been described that these accumulate many heavy metals 65-67.
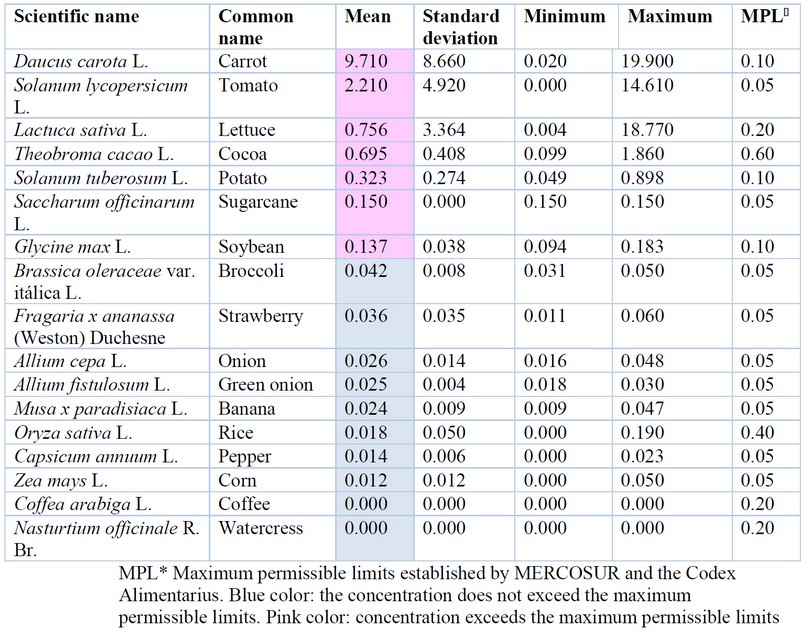
Table 3. Cadmium concentration in vegetables from Ecuador and its comparison with the maximum permissible limits (MPL).
High concentrations were also observed in tomato (Solanum lycopersicum) (2.21 mg/kg Cd) and lettuce (Lactuca sativa) (0.76 mg/kg Cd) analyzed in Quito. However, these same vegetables obtained in an organic fair presented very high values (atypical) of 7.31 mg/kg and 14.61 mg/kg Cd for tomato and 9.39 mg/kg and 18.77 mg/kg Cd for lettuce 54, a worrying fact because organic food is assumed to be healthier. Another food of great importance for Ecuador that exceeded the limits was cocoa (Theobroma cacao), which presented high Cd contents in the almond (0.695 ± 0.408 mg/kg); however, the Codex Alimentarius has not determined the MPL for cocoa beans; nonetheless, it slightly exceeds the MPL determined by the European Union 52 of 0.6 mg/kg Cd for cocoa powder with an average of 0.9 mg/kg of Cd 61 in Ecuadorian cocoa and attributed this value to the capacity of cocoa plants to accumulate high concentrations of Cadmium and to its cultivation in young soils. On the other hand, processed cocoa powder showed higher values than cocoa beans in a cocoa commercial brand 54. Values below the standard in the cocoa powder were found 62, with 0.236±0.082 mg/kg Cd for Brand 1 and 0.169±0.066 mg/kg for Brand 2; however, Brand 3 presented 1.44 ±0.212 mg/kg, a much higher value than that found in the almond, which shows that there may be postharvest contamination. It is important to note that cocoa drinks are consumed mainly by children, increasing the risk of suffering from any diseases generated by excess Cd. These results indicate that the postharvest handling machinery, inputs, and additives could generate heavy metal contamination in the final product for processed or highly processed products.
Figure 1B shows a dendrogram composed of three large groups: the one with a maximum accumulation of Cd represented by Daucus carota; a second group that exceeds the MPL, made up of Glycine max, Lactuca sativa, Saccharum officinarum, Solanum lycopersicum, Solanum tuberosum and Theobroma cacao; and the third group of foods suitable for consumption since they present MPL values below the Codex Alimentarius: Brassica oleracea var. italica, Allium fistulosum, Fragaria x ananassa, Allium cepa, Oryza sativa, Capsicum annuum, Zea mays and Nasturtium officinale.
Among the possible causes of high Cd values in food is natural contamination: contamination of soils by geographical origin 55, or because of the deposition of ash and volcanic material 64. However, there is a greater probability that the contamination of agricultural soils presents anthropogenic contamination due to the use of fertilizers, especially phosphates that, in Ecuador, contain up to 41.30 ± 1.65 mg/kg of Cd, exceeding 41 times the limits recommended by the Washington State Ecology Department 65. It has also been shown that there is contamination by heavy metals in river water used for irrigation of crops 66-68, contaminated healthy water and wastewater. In addition, another critical factor is the proximity to roads and populated areas. In this sense, a correlation has been found between the Cd content in the rice cultivation soil and the distance to the roads 65.
Mercury (Hg) presence in food products
Mercury was the metal least studied for its occurrence in vegetables in Ecuador; there is only one study in Solanum tuberosum49 collected in farms in the Aloasí-Pichincha canton. According to the European Union, an average of 0.04 mg/kg Hg was determined, which exceeds the MPL of 0.01 mg/kg Hg. The Hg content evaluated in Solanum tuberosum exceeded 4 times the MPL; this value is similar to the maximum values evidenced in foods produced in areas contaminated by coal mines for leaf vegetables (0.06 mg/kg), fruit vegetables (0.06 mg/kg) and grains (0.04 mg/kg) 61. In contaminated soils, up to 20 mg/kg of Hg has been quantified in this vegetable 70. One possible explanation is the contamination of agricultural soils. There is evidence that the Hg content in cultivation soils is mainly due to atmospheric depositions and the use of fertilizers 63; these depositions can come from anthropogenic and natural sources.
Within the natural origins, it has been determined that volcanoes constitute a significant source of Hg to the surrounding environment 64. Atmospheric depositions can also contribute to the Hg content in the soil.
This confirms the need to know the characteristics of the crop soils to determine their contribution to pollution, in addition to considering the influence of volcanoes and the industrial activities of the nearest cities. It is recommended that the authorities and universities carry out more studies to determine mercury in foods of plant origin and consider carrying out studies in mining areas where mercury levels are high in the rivers near the plantations.
Lead (Pb) detected in products of plant origin
Regarding the analysis of the presence of Pb in foods of plant origin, 6 of the 16 foods studied exceeded the maximum permissible limits according to Codex Alimentarius and MERCOSUR (Table 4). The highest concentration was evidenced in Saccharum officinarum from La Troncal-Guayas 65 with 4.32 mg/kg Pb, a quantity that exceeds the MPL of 0.10 mg/kg Pb for sugars 56. Sugarcane showed the highest Pb values, with 43.2 times the MPL, a value higher than that reported before 66 (0.01 - 1.11 mg/kg) in the sugarcane stem in India. It is important to note that Saccharum officinarum can accumulate high concentrations of heavy metals, and therefore it can be considered a potential phytoremediator of Cd and Pb 67–78.
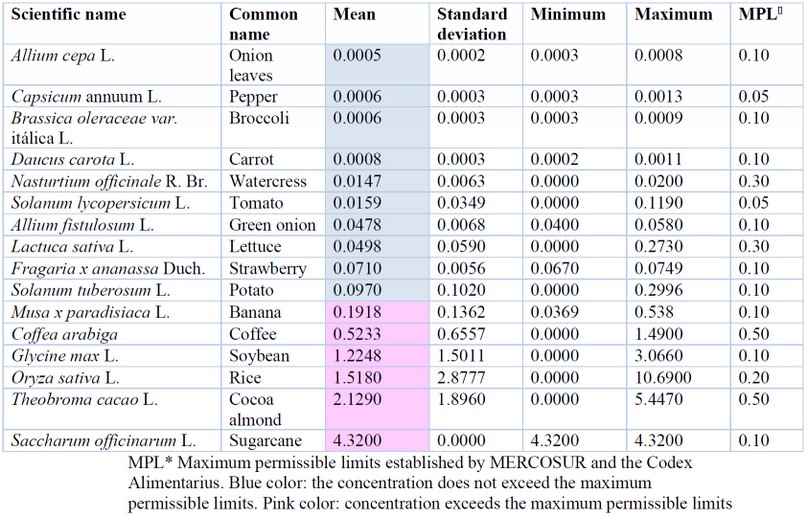
Table 4. Lead concentration in vegetables from Ecuador
Likewise, Theobroma cacao, studied in the provinces of Los Ríos and Manabí 79,80 obtained an average of 2.38 mg/kg Pb, which exceeds the MPL of 0,50 mg/kg Pb 49.
The Pb content present in Oryza sativa, studied in the provinces of Azuay 73 and Guayas 82 was 1.52 mg/kg, which exceeded the MPL of 0.2mg/kg 49,61. Notably, atypical values were evidenced in the cantons of Nobol (10.69 and 5.65 mg/kg) and Daule (10.10 mg/kg) belonging to the province of Guayas82. This research demonstrated that lead contamination was due to the high bioavailable levels of this metal in cultivated soils and the proximity to the road network 82. Likewise, critical points for lead contamination have been established due to vehicle emissions 75. Rice contamination is considered very serious because it is part of many typical dishes of the region, and there is a high consumption nationwide, increasing the intake of this metal and the possibility of suffering from diseases generated by Pb.
The results obtained for Glycine max L. were 1.22 mg/kg in different markets of Guayaquil 84, with the LMP being 0.1 mg/kg Pb 53. Likewise, coffee (Coffea sp.) obtained an average value of 0.52 mg/kg Pb77, which is above the MPL (0.50 mg/kg Pb) established by MERCOSUR (2011). In another investigation, the same authors established a relationship between the concentration found in the soil and in the coffee 77, the source of the contamination being the naturally contaminated soil in the province of Loja. Fortunately, they indicated that Pb was not detectable in coffee infusion, so it does not represent a danger to consumers.
On the other hand, in bananas (Musa x paradisiaca), concentration ranges between 0.0369-0.538 mg/kg of Pb 78. Likewise, these authors found differences between provinces; the highest results were from the samples collected in the provinces of Bolívar and Santa Elena, which had 0.420 mg/kg and 0.538 mg/kg of Pb, respectively; on the contrary, the lowest concentrations in banana were found in the province of Azuay with 0.0369 mg/kg Pb 78.
Figure 1C shows a dendrogram where 3 groups of plants with different degrees of contamination can be appreciated: the first one is represented by the species Saccharum officinarum with the highest accumulation of Pb; the second consists of species that exceed the permissible limits: Glycine max, Oryza sativa, Theobroma cacao and Coffea arabiga; and a third group made up of species suitable for consumption, which do not exceed the permissible limits: Allium cepa, Capsicum annuum, Brassica oleraceae var. itálica, Daucus carota, Nasturtium officinale, Solanum lycopersicum, Allium fistulosum, Lactuca sativa, Fragaria x ananassa and Solanum tuberosum.
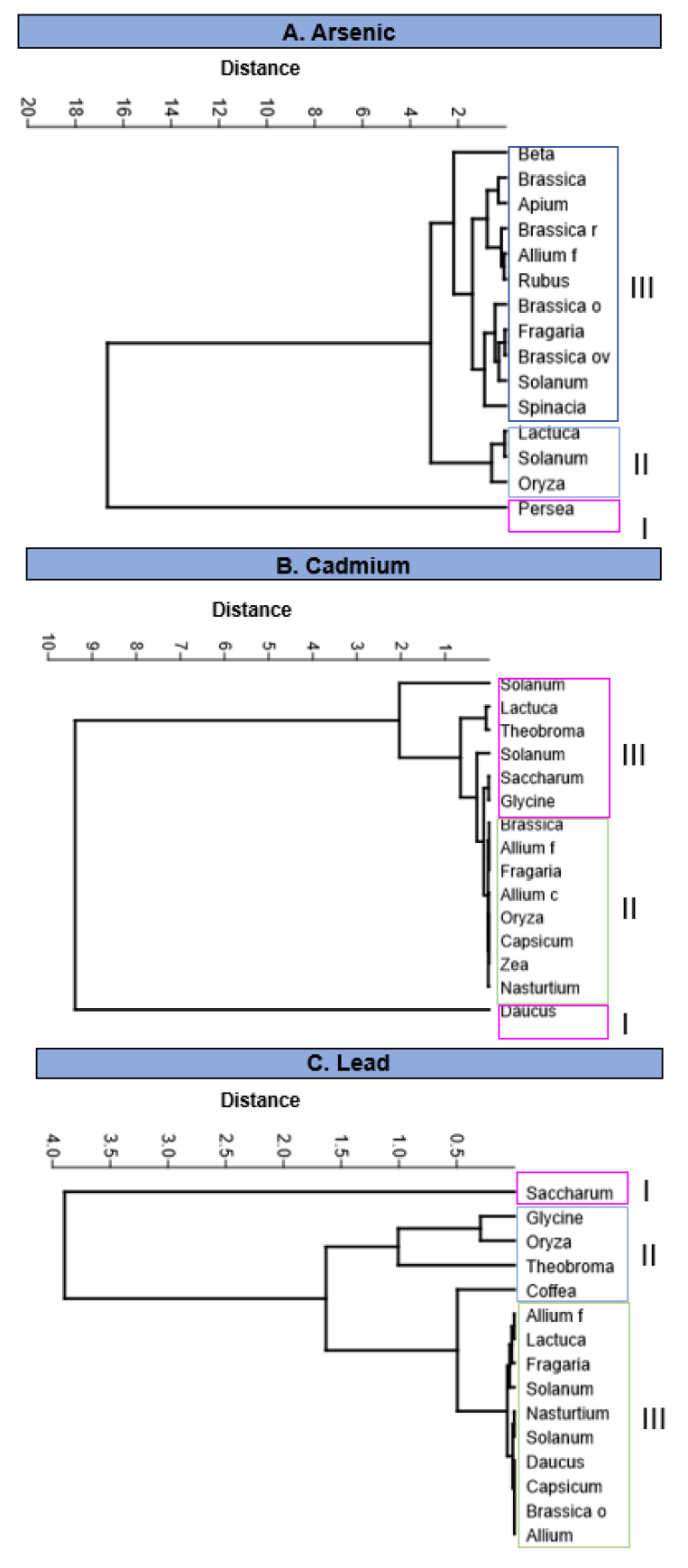
Figure 1. Cluster dendrogram of plant food species that accumulate arsenic (As), Cadmium (Cd) and lead (Pb), based on the UPGMA algorithm and the Euclidean distance between the concentrations observed by species.
One of the sources of crop contamination by Pb and Cd are the agrochemicals used. Several studies have reported that the excessive use of fertilizers (phosphate and nitrogenous) and manure of animal origin for an extended period can cause the accumulation of Cd and Pb in the soil and the plant 79,80.
Another important aspect is the location of the crop since it has been shown that urban soils contain a more significant amount of Pb 65. It is essential to consider that the vegetables grown in urban gardens present high concentrations of Pb, increasing the risk of suffering diseases in consumers, and therefore, it is recommended to carry out monitoring of urban gardens because the levels of Pb in the soil are very high. 89.
In sum, the factors that influence the content of heavy metals in vegetables are irrigation with contaminated water, the addition of fertilizers and pesticides, industrial emissions, transportation, the harvesting process, storage and the method of sale 90.
Regarding the distribution of metals in vegetables in Ecuador, Figure 2 shows that the foods with the highest average content of As, Cd and Pb, are located in the province of Tungurahua, Orellana and Guayas, respectively. The highest average concentrations of As in vegetables that occurred in the province of Tungurahua (4.772 mg/kg) could be influenced by volcanic activity; while Cd presented higher concentrations in Zamora Chinchipe (1.050 mg/kg), where mining and agricultural activity takes place. In the province of Guayas and Los Ríos, high concentrations of vegetables were evidenced with 2.520 and 2.170 mg/kg Pb, respectively, where contamination could be due to industrial activity.
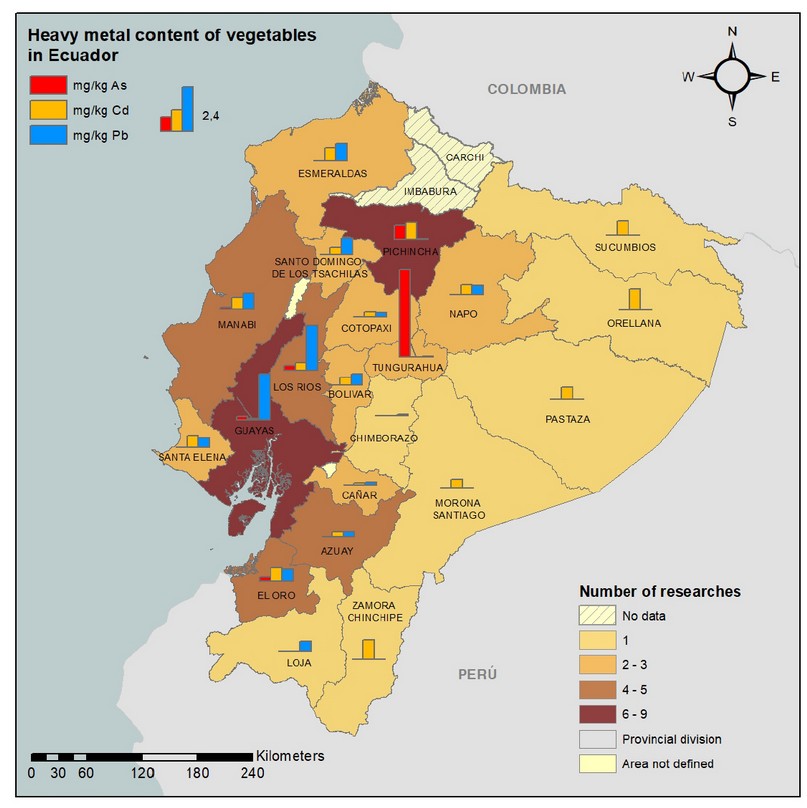
Figure 2. Distribution of foods of plant origin contaminated with heavy metals in Ecuador. The colors of the provinces correspond to the number of studies carried out and the bars to the concentrations of As (red), Cd (yellow) and Pb (blue).
Likewise, it was observed that the most significant number of research works had been carried out in the provinces of Guayas and Pichincha, as they are the country's main provinces; meantime, the heavy metal content in the Carchi and Imbabura provinces is yet unknown.
As seen in Figure 3, there are species capable of accumulating several metals/metalloids simultaneously, which could generate synergy and a greater toxic effect than that recorded individually. The species that accumulated the three elements (As, Cd and Pb) were B. oleracea (with the prevalence of As), Fragaria x ananassa, L. sativa, and S. tuberosum accumulated four metals since Hg was also found. It is essential to mention that the works analyzed in this study determined the total concentrations of heavy metals; although, to calculate the risk associated with exposure, it is necessary to know what percentage of these elements are bioaccessible 91. Only one report 92 determined gastric bio-accessibility in chocolate raw material (cocoa beans and liqueurs) showing that almost 100% of the total Cd content is bio-accessible after ingestion, which represents a risk for consumers. Therefore, it is proposed to monitor foods in which heavy metals were measured and determine the real risk to the population's health; additionally, the total amount and the bio-accessibility level in foods of plant origin in Ecuador must be established.
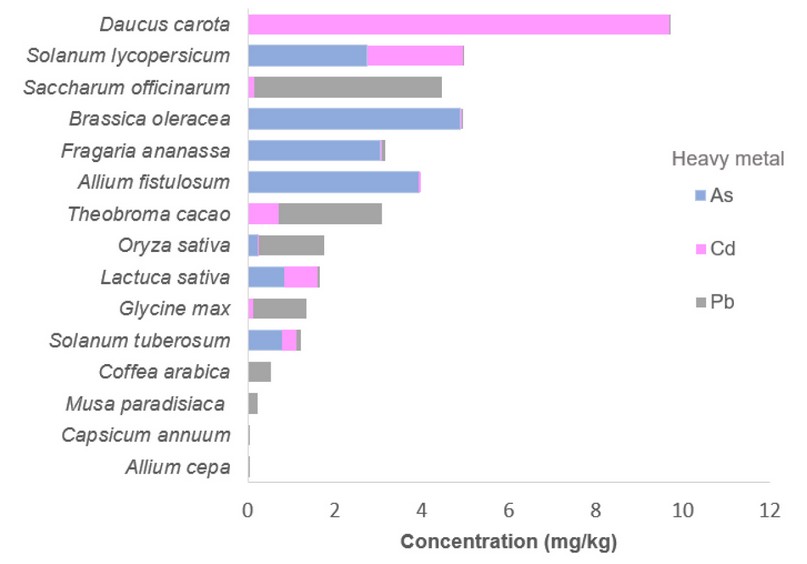
Figure 3. Evidence of multi-metal contamination in vegetables from Ecuador.
Ecuador is a country with a high incidence of cancer, and it has been estimated that the cases would increase according to a study 45; one of the sources of carcinogenic agents is vegetables, which is why it was proposed to the authorities:
- Monitor all foods of plant origin from the field to the consumer's plate, considering the province of production and possible sources of heavy metals.
- Ensure compliance with INEN and Codex Alimentarius standards is mandatory for foods for internal consumption in Ecuador to ensure food safety for Ecuadorians.
- Farmers are advised to use heavy metal-free fertilizers, measure metal levels in their soils before sowing seeds, and choose plant varieties with a lower bioaccumulation rate of these items.
CONCLUSIONS
This research aimed to construct a baseline of contamination by heavy metals in foods of plant origin in Ecuador. Our research found that there is contamination in avocado, cocoa, potatoes, carrots, sugar cane, rice and soybeans, which represents a risk to the health of Ecuadorians. The genera with the highest concentration of arsenic were Persea, in the case of Cadmium Daucus and lead Saccharum.
In addition, it was shown that there are several metals with simultaneous risen levels in the same food, representing a risk due to multi-metal contamination and the synergy of metals, and their possible impacts on the population's health should be studied. On the other hand, the lack of studies about mercury contamination in food in Ecuador was evidenced. In addition, there are very few studies in the Ecuadorian Amazon and none in Imbabura and Carchi, so we recommended analyzing plant products from these areas of Ecuador.
The authorities are called upon to carry out a nationwide monitoring study about heavy metal contents in food to protect the population's health. Unfortunately, the contamination of agricultural soils is increasing, together with the risk of increasing the levels of heavy metals in agricultural products.
REFERENCES
1. Ali H, Khan E, Ilahi I. Environmental chemistry and ecotoxicology of hazardous heavy metals: Environmental persistence, toxicity, and bioaccumulation. J Chem. 2019; Available from: https://doi.org/10.1155/2019/6730305
2. Khanam R, Kumar A, Nayak AK, Shahid M, Tripathi R, Vijayakumar S, et al. Metal(loid)s (As, Hg, Se, Pb and Cd) in paddy soil: Bioavailability and potential risk to human health. Sci Total Environ [Internet]. 2020;699:134330. Available from: https://doi.org/10.1016/j.scitotenv.2019.134330
3. Kumar P, Soo S, Zhang M, Fai Y, Kim K. Heavy metals in food crops: Health risks, fate, mechanisms, and management. Environment International. 2019;125:365-385. https://doi.org/10.1016/j.envint.2019.01.067
4. Smith A, Lingas E, Rahman M. Contamination of drinking-water by arsenic in Bangladesh: a public health emergency: RN - Bull. WHO, v. 78, p. 1093-1103. Bull World Health Organ. 2000;78(9):1093–103.
5. Oberoi S, Barchowsky A, Wu F. The global burden of disease for skin, lung, and bladder cancer caused by arsenic in food. Cancer Epidemiol Biomarkers Prev. 2014;23(7):1187–94.
6. Rahman MA, Rahman MM, Reichman SM, Lim RP, Naidu R. Heavy metals in Australian grown and imported rice and vegetables on sale in Australia: Health hazard. Ecotoxicol Environ Saf [Internet]. 2014;100(1):53–60. Available from: http://dx.doi.org/10.1016/j.ecoenv.2013.11.024
7. Zavala YJ, Duxbury JM. Arsenic in rice: I. Estimating normal levels of total arsenic in rice grain. Environ Sci Technol. 2008;42(10):3856–60.
8. Batista BL, Nacano LR, Freitas R de, Oliveira-Souza VC de, Barbosa F. Determination of Essential (Ca, Fe, I, K, Mo) and Toxic Elements (Hg, Pb) in Brazilian Rice Grains and Estimation of Reference Daily Intake. Food Nutr Sci. 2012;03(01):129–34.
9. Corguinha APB, Souza GA, Goncalves VC, Carvalho CA, Lima WE, Martins FA, et al. Assessing arsenic, Cadmium, and lead contents in major crops in Brazil for food safety purposes. J Food Compos Anal. 2015;37:143–50.
10. Corguinha APB, Gonçalves VC, de Souza GA, de Lima WEA, Penido ES, Pinto CABP, et al. Cadmium in potato and soybeans: Do phosphate fertilization and soil management systems play a role? J Food Compos Anal. 2012;27(1):32–7.
11. Mandal BK, Suzuki KT. Arsenic round the world: A review. Vol. 58, Talanta. Elsevier; 2002. p. 201–35.
12. Maiti S, Chattopadhyay S, Deb B, Samanta T, Maji G, Pan B, et al. Antioxidant and metabolic impairment result in DNA damage in arsenic-exposed individuals with severe dermatological manifestations in Eastern India. Environ Toxicol. 2012 May;27(6):342–50.
13. Cameán A, Repetto M, editors. Toxicología Alimentaria. Madrid - Buenos Aires; 2006.
14. Reyes YC, Vergara I, Torres OE, Díaz M, González EE. Contaminación por metales pesados: implicaciones en salud, ambiente y seguridad alimentaria. Rev Ing Investig y Desarro. 2016;16(2):66–77.
15. Bernhoft RA. Cadmium toxicity and treatment. Sci World J. 2013;2013.
16. Satarug S, Garrett SH, Sens MA, Sens DA. Cadmium, environmental exposure, and health outcomes. Environ Health Perspect. 2010;118(2):182–90.
17. García P, Esmeralda P, Cruz A, Isabel M. Los efectos del cadmio en la salud. Rev Espec Médico-Quirúrgicas. 2012;17(3):199–205.
18. Flora S, Agrawal S. Arsenic, Cadmium, and Lead. In: Gupta R, editor. Reproductive and Developmental Toxicology. 2nd ed. Elsevier Inc.; 2017. p. 537–66.
19. Jacobo-Estrada T, Santoyo-Sánchez M, Thévenod F, Barbier O. Cadmium handling, toxicity and molecular targets involved during pregnancy: Lessons from experimental models. Int J Mol Sci. 2017;18(7).
20. Järup L. Cadmium overload and toxicity. Nephrol Dial Transplant. 2002;17(suppl_2):35–9.
21. Hamid Y, Tang L, Sohail MI, Cao X, Hussain B, Aziz MZ, et al. An explanation of soil amendments to reduce cadmium phytoavailability and transfer to food chain. Sci Total Environ [Internet]. 2019;660:80–96. Available from: https://doi.org/10.1016/j.scitotenv.2018.12.419
22. Satarug S, Vesey DA, Gobe GC. Current health risk assessment practice for dietary Cadmium: Data from different countries. Food Chem Toxicol [Internet]. 2017;106(June):430–45. Available from: http://dx.doi.org/10.1016/j.fct.2017.06.013
23. Driscoll CT, Mason RP, Chan HM, Jacob DJ, Pirrone N. Mercury as a global pollutant: Sources, pathways, and effects. Environ Sci Technol. 2013;47(10):4967–83.
24. Mitchell CPJ, Gilmour CC. Methylmercury production in a Chesapeake Bay salt marsh. J Geophys Reseach. 2008;113(August):1–14.
25. León D, Peñuela G. Trascendencia del metilmercurio en el ambiente, la alimentación y la salud humana. Prod + Limpia. 2011;6(2):108–16.
26. Rice KM, Jr EMW, Wu M, Gillette C, Blough ER. Environmental Mercury and Its Toxic Effects. J Prev Med Public Heal. 2014;47:74–83.
27. Zheng N, Wang Q, Zheng D. Health risk of Hg, Pb, Cd, Zn, and Cu to the inhabitants around Huludao Zinc Plant in China via consumption of vegetables. Sci Total Environ. 2007;383:81–9.
28. Rasmussen P, Mierle G. The analysis of vegetation for total mercury. Water Air Soil Pollut. 1991;56:379–90.
29. Ghasemidehkordi B, Akbar A, Nazem H, Fazilati M. Concentration of lead and mercury in collected vegetables and herbs from Markazi province, Iran : a non-carcinogenic risk assessment. Food Chem Toxicol [Internet]. 2018;113(November 2017):204–10. Available from: https://doi.org/10.1016/j.fct.2018.01.048
30. Rubio C, Gutiérrez A., Martín Izquierdo R., Revert C, Lozano G, Hardisson A. El plomo como contaminante alimentario. Rev Toxicol. 2004;21(2–3).
31. Selbst S. Envenenamiento por plomo en los niños. Arch pediatr Urug. 2001;72:38–44.
32. Markowitz M. Lead poisoning: A disease for the next millennium. Curr Probl Pediatr. 2000;30(3):62–70.
33. Mushak P. Lead and Public Health: Science, Risk and Regulation [Internet]. Elsevier; 2011 [cited 2015 Feb 12]. 950 p. Available from: https://books.google.com/books?id=T5HcahQSOfAC&pgis=1
34. Martin S, Griswold W. Human Health Effects of Heavy Metals. Environ Sci Technol Briefs Citizens. 2016;15:1–6.
35. Li F, Liu C, Yang Y, Bi X, Liu T, Zhao Z. Natural and anthropogenic lead in soils and vegetables around Guiyang City, southwest China: A Pb isotopic approach. Sci Total Environ [Internet]. 2012;431:339–47. Available from: http://dx.doi.org/10.1016/j.scitotenv.2012.05.040
36. Fu W, Ma G. The characters and health risk assessment of vegetable Pb in Jilin Suburb. Procedia Environ Sci [Internet]. 2013;18:221–6. Available from: http://dx.doi.org/10.1016/j.proenv.2013.04.028
37. Agricultura, la base de la economía y la alimentación. Available online: https://www.agricultura.gob.ec/agricultura-la-base-de-la-economia-y-la-alimentacion/ (Accessed on 9 may 2022)
38. Ministerio de Agricultura y Ganadería. Resumen Ejecutivo de los Diagnósticos Territoriales del Sector Agrario. 2020. Available online: https://www.agricultura.gob.ec/wp-content/uploads/2020/08/Resumen-Ejecutivo-Diagn%C3%B3sticos-Territoriales-del-Sector-Agrario_14-08-2020-1_compressed.pdf (accessed on 9 may 2022)
39. Counter SA, Buchanan LH, Ortega F, Chiriboga R, Correa R, Collaguaso MA. Lead Levels in the Breast Milk of Nursing Andean Mothers Living in a Lead-Contaminated Environment. J Toxicol Environ Heal Part A [Internet]. 2014;77(February 2015):993–1003. Available from: http://www.tandfonline.com/doi/abs/10.1080/15287394.2014.897281
40. Rivera K, Pernía B. Determinación de los niveles de plomo en sangre en trabajadores de fábricas de baterías ubicadas en Guayaquil-Ecuador. Enfoque UTE. 2021;12(2):1–18.
41. Counter, S. A. Neurocognitive effects of chronic lead intoxication in Andean children. J. Neurol. Sci. 1998; 160, pp. 47–53.
42. Counter, S. A.; Buchanan, L. H. Counter and Buchanan Neuro-Ototoxicity in Andean Adults With Chronic Lead and Noise Exposure. JOEM, 2002. 44, no. 1, pp. 30–38.
43. Armijos, R. X.; Weigel, M. M.; Obeng-gyasi, E.; Racines-Orbe M. Elevated blood lead and metal / metalloid levels and environmental exposure sources in urban Ecuadorian school-age children and mothers, Int. J. Hyg. Environ. Health, 2021, vol. 235, no. November 2020, p. 113770, doi: 10.1016/j.ijheh.2021.113770.
44. OMS, Globoscan. 2020. Cancer in Ecuador. Available online: https://gco.iarc.fr/today/data/factsheets/populations/218-ecuador-fact-sheets.pdf (accessed on 9 may 2022)
45. Lin, Y.; Zheng, Y.; Wang, H.; Wu, J. Global Patterns and Trends in Gastric Cancer Incidence Rates (1988-2012) and Predictions to 2030, Gastroenterology, 2021, vol. 161, no. 1, pp. 116-127.e8, 2021, doi: 10.1053/j.gastro.2021.03.023.
46. DeSantis, C. E.; Bray, F.; Ferlay, J.; Lortet-tieulent, J.; Anderson, B. O.; Jemal, A. International Variation in Female Breast Cancer Incidence and Mortality Rates, Cancer Epidemiol Biomarkers Prev, 2012, vol. 24, no. 10, pp. 8–12, doi: 10.1158/1055-9965.EPI-15-0535.
47. Fiallos M, Arancibia M. Cuantificación de metales pesados y calidad microbiológica de frutas y vegetales que se expenden en el mercado mayorista de la ciudad de Ambato - Ecuador. Universidad Técnica de Ambato; 2017.
48. MERCOSUR. Reglamento Técnico MERCOSUR sobrel límites máximos de contaminantes inorgánicos en alimentos. 2011;(1):1–18.
49. Castillo R. Determinación de metales pesados procedentes del proceso eruptivo del volcán Cotopaxi en pasto (Cynodon dactylon) y en los cultivos de papa (Solanum tuberosum), de la zona ganadera de Machachi. Universidad de las Fuerzas Armadas; 2019.
50. Moreno E. Evaluación de la presencia de arsénico en arroz sin cáscara producido en Ecuador. Universidad Tecnológica Equinoccial; 2018.
51. Bundschuh J, Nath B, Bhattacharya P, Liu CW, Armienta MA, Moreno López M V., et al. Arsenic in the human food chain: The Latin American perspective. Sci Total Environ. 2012;429:92–106.
52. Cumbal L, Vallejo P, Rodriguez B, Lopez D. Arsenic in geothermal sources at the north-central Andean region of Ecuador: Concentrations and mechanisms of mobility. Environ Earth Sci. 2010;61(2):299–310.
53. FAO, OMS. Norma general para los contaminantes y las toxinas presentes en los alimentos y piensos. 1995 p. 76.
54. Coronel E, Mastrocola N. Determinación de metales pesados plomo (Pb) y cadmio (Cd) en hortalizas de consumo directo producidas orgánicamente. Universidad Central del Ecuador; 2018.
55. Shaheen N, Irfan NM, Khan IN, Islam S, Islam MS, Ahmed MK. Presence of heavy metals in fruits and vegetables: Health risk implications in Bangladesh. Chemosphere. 2016;152:431–8.
56. Zhou H, Yang WT, Zhou X, Liu L, Gu JF, Wang WL, et al. accumulation of heavy metals in vegetable species planted in contaminated soils and the health risk assessment. Int J Environ Res Public Health. 2016;13(3).
57. Fytianos K, Katsianis G, Triantafyllou P, Zachariadis G. Accumulation of Heavy Metals in Vegetables Grown in an Industrial Area in Relation to Soil. Bull Environ Contam Toxicol. 2001;67(3):0423–30.
58. Sultana MS, Rana S, Yamazaki S, Aono T, Yoshida S. Health risk assessment for carcinogenic and non-carcinogenic heavy metal exposures from vegetables and fruits of Bangladesh. Cogent Environ Sci. 2017;3(1).
59. Muñoz O, Diaz OP, Leyton I, Nuñez N, Devesa V, Súñer MA, et al. Vegetables collected in the cultivated Andean area of Northern Chile: Total and inorganic arsenic contents in raw vegetables. J Agric Food Chem. 2002;50(3):642–7.
60. Comisión Alimentaria Unión Europea. Reglamento (CE) N° 1881/2006: Contenido máximo de determinados contaminantes en los productos alimenticios. 2006.
61. Argüello D, Chavez E, Lauryssen F, Vanderschueren R, Smolders E, Montalvo D. Soil properties and agronomic factors affecting cadmium concentrations in cacao beans: A nationwide survey in Ecuador. Sci Total Environ [Internet]. 2019;649:120–7. Available from: https://doi.org/10.1016/j.scitotenv.2018.08.292
62. Sánchez-Soledispa CE, Zambrano-vera DC, Arévalo-castro O, Pernía B. Baseline study of heavy metal concentrations in powdered chocolate marketed in Guayaquil, Ecuador. Investigatio. 2021;(16):62–77.
63. Pozo W, Sanfeliu T, Carrera G. Metales pesados en humedales de arroz en la cuenca baja del río Guayas. MASKANA. 2011;2(1):17–30.
64. Hall ML, Samaniego P, Le Pennec JL, Johnson JB. Ecuadorian Andes volcanism: A review of Late Pliocene to present activity. J Volcanol Geotherm Res. 2008;176(1):1–6.
65. Muñoz J, Pernía B, Mero M, Larreta E, Bravo K, Zambrano J, et al. Determination of Cadmium in fertilizers, agricultural soils and rice in the province of Guayas-Ecuador. Acta Agron. 2020; In Press.
66. Carling GT, Diaz X, Ponce M, Perez L, Nasimba L, Pazmino E, et al. Particulate and dissolved trace element concentrations in three southern Ecuador rivers impacted by artisanal gold mining. Water Air Soil Pollut. 2013; 224:1415.
67. Mero M, Pernía B, Ramírez-Prado N, Bravo K, Ramírez L, Larreta E, et al. Concentración de cadmio en agua, sedimentos, Eichhornia crassipes y Pomacea canaliculata en el Río Guayas (Ecuador) y sus afluentes. Rev Int Contam Ambient [Internet]. 2019;35(3):623–40. Available from: https://www.revistascca.unam.mx/rica/index.php/rica/article/view/RICA.2019.35.03.09/46875
68. Ramírez-prado N, Pernía B, Mero M, Larreta E, Noboa-cárdenas A, Ramírez-moreira L, et al. Pomacea canaliculata (Lamarck, 1828) como bioindicador de la presencia de cadmio en el río Guayas, Daule y Babahoyo. Rev Cient Cien Nat Ambien. 2016;10(1):19–28.
69. Li R, Wu H, DIng J, Fu W, Gan L, Li Y. Mercury pollution in vegetables, grains and soils from areas surrounding coal-fired power plants. Sci Rep. 2017;7(March):1–9.
70. Kimakova T, Poracova J. Mercury content in selected organs of potato (Solanum tuberosum) Plants in the areas with the elevated mercury soil content in Slovakia. Epidemiology. 2011;22(1):13–299.
71. Wang Q, Zhang J, Xin X, Zhao B, Ma D, Zhang H. The accumulation and transfer of arsenic and mercury in the soil under a long-term fertilization treatment. Vol. 16, Journal of Soils and Sediments. 2016.
72. Martin RS, Witt MLI, Sawyer GM, Thomas HE, Watt SFL, Bagnato E, et al. Bioindication of volcanic mercury (Hg) deposition around Mt. Etna (Sicily). Chem Geol. 2012;310–311:12–22.
73. Alcívar M, Pernía B. Determinación de cadmio y plomo en productos derivados de la caña: azúcar blanca, morena y panela, comercializados en Ecuador. In: V Congreso Iberoamericano sobre Ambiente y Sustentabilidad. Manabí: Universidad Estatal del Sur de Manabí; 2019. p. 14–5.
74. Pandey B, Suthar S, Singh V. Accumulation and health risk of heavy metals in sugarcane irrigated with industrial effluent in some rural areas of Uttarakhand, India. Process Saf Environ Prot. 2016;102:655–66.
75. Yan Z, Xia H. Evaluation of the phytoremediation potential of sugarcane for metal-contaminated soils. 2010 4th Int Conf Bioinforma Biomed Eng iCBBE 2010. 2010;
76. Yadav D, Jain R, Rai R. Impact of heavy metals on sugarcane. In: Sherameti I, Varma A, editors. Soil Heavy Metals. 2010. p. 1–18.
77. Xia H, Chi X, Cheng W. Uptake and growth response of Saccharum officinarum to lead pollution in soil. In: 2009 3rd International Conference on Bioinformatics and Biomedical Engineering. IEEE; 2009. p. 1–4.
78. Xia H, Yan Z, Chi X, Cheng W. Evaluation of the phytoremediation potential of Saccharum officinarum for Cd-contaminated soil. 2009 Int Conf Energy Environ Technol ICEET 2009. 2009;3(c):314–8.
79. Días L, Mendoza E, Bravo Bustamante M, Domínguez N. Determinación de Cadmio y Plomo en almendras de cacao (Theobroma cacao), proveniente de fincas de productores orgánicos del cantón Vinces. Espirales. 2018;2(15).
80. Intriago F, Talledo M, Cuenca G, Macías J, Álvarez J, Menjívar J. Evaluación del contenido de metales pesados en almendras de cacao (Theobroma cacao L.) durante el proceso de beneficiado. PRO-SCIENCES Rev Prod Ciencias e Investig. 2019;3(1881):17–23.
81. Huiracocha J. Evaluación del riesgo toxicológico por cadmio y plomo en granos de arroz (Oryza sativa) comercializados en la ciudad de Cuenca. Universidad de Cuenca; 2018.
82. Sinchi I, Pernía B. Determinación de plomo en suelo y arroz (Oryza sativa L.) en dos cantones de la Provincia del Guayas. Universidad de Guayaquil; 2019.
83. Bedregal P, Ubillús M, Hurtado J, Mendoza P. Evaluación de la contaminación atmosférica en puntos críticos de la ciudad de Lima y Callao, utilizando Tillandsia capillaris como biomonitor de la ciudad de Lima y Callao. Inf Científico Tecnológico. 2012;(January 2014):41–7.
84. López D, Pernía B. Determinación de cadmio y plomo en soya (Glycine max L.) en grano, en polvo y líquida comercializada en la ciudad de Guayaquil-Ecuador. In: V Congreso Iberoamericano sobre Ambiente y Sustentabilidad. Universidad Estatal del Sur de Manabí; 2019. p
85. Viñan J, Pernía B. Determinación de plomo en café Industrial y Artesanal comercializados en la Provincia de Loja. In: V Congreso Iberoamericano sobre Ambiente y Sustentabilidad. Universidad Estatal del Sur de Manabí; 2019. p. 14–5.
86. Romero-Estévez, D.; Yánez-Jácome, G. S.; Simbaña-Farinango, K.; Navarrete, H. Distribution, contents, and health risk assessment of Cadmium, lead, and nickel in bananas produced in Ecuador. 2019. Foods, 8(8):330. https://doi.org/10.3390/foods8080330
87. Bai LY, Zeng XB, Su SM, Duan R, Wang YN, Gao X. Heavy metal accumulation and source analysis in greenhouse soils of Wuwei District, Gansu Province, China. Environ Sci Pollut Res. 2015;22(7):5359–69.
88. AlKhader AM. The impact of phosphorus fertilizers on heavy metals content of soils and vegetables grown on selected farms in Jordan. Agrotechnology. 2015;05(01).
89. Frank JJ, Poulakos AG, Tornero-Velez R, Xue J. Systematic review and meta-analyses of lead (Pb) concentrations in environmental media (soil, dust, water, food, and air) reported in the United States from 1996 to 2016. Sci Total Environ [Internet]. 2019;694:133489. Available from: https://doi.org/10.1016/j.scitotenv.2019.07.295
90. Pan XD, Wu PG, Jiang XG. Levels and potential health risk of heavy metals in marketed vegetables in Zhejiang, China. Sci Rep. 2016;6:1–7.
91. Upadhyay MK, Shukla A, Yadav P, Srivastava S. A review of arsenic in crops, vegetables, animals and food products. Food Chem [Internet]. 2019;276:608–18. Available from: https://doi.org/10.1016/j.foodchem.2018.10.069
92. Barraza F, Schreck E, Leveque T, Uzu G, López F, Ruales J, et al. Cadmium bioaccumulation and gastric bioaccessibility in cacao: A field study in areas impacted by oil activities in Ecuador. Environ Pollut J. 2017;229:950–63.
93. Mantilla R, Mastrocola N. Determinación de metales pesados y pérdidas poscosecha en dos hortalizas de consumo directo pimiento (Capsicum annuum) y cebolla (Allium cepa) [Internet]. Universidad Central del Ecuador; 2018. Available from: http://www.uce.edu.ec
94. Tonato E, Briceño J. Determinación de metales en cebolla de rama (Allium fistulosum L.), cultivada en suelos agrícolas cercanos al volcán Tungurahua. Universidad Técnica de Ambato; 2019.
95. Escobar S. Determinación de la presencia de plomo y cadmio en frutilla (Fragaria ananassa) y tomate (Solanum lycopersicum) en el Quinche [Internet]. Vol. 9. Universidad Central del Ecuador; 2016. Available from: http://www.dspace.uce.edu.ec/bitstream/25000/10068/1/T-UCE-0004-81.pdf
96. Carrillo G, Valdés L. Determinación microbiológica y de metales pesados en lechuga de repollo (Lactuca sativa), expendidos en los diferentes mercados del Distrito Metropolitano de Quito [Internet]. Universidad Politécnica Salesiana Sede Quitois; 2016. Available from: http://dspace.ups.edu.ec/bitstream/123456789/5081/1/UPS-CYT00109.pdf
97. Velasquez M, Mastrocola N. Determinación de metales pesados y pérdidas poscosecha en dos hortalizas de consumo directo: tomate (Solanum lycopersicum) y lechuga (Lactuca sativa) [Internet]. Universidad Central del Ecuador; 2017. Available from: http://www.dspace.uce.edu.ec/handle/25000/12691
98. Villegas N, Moreno I, Zavala A, Guevara P. Prevalencia de niveles detectables de plomo y cromo en hortalizas de consumo directo en la alimentación recolectadas en zonas agrícolas de Riobamba. Rev Caribeña Ciencias Soc. 2018;36(2018–10):1–21.
99. Torres N, Pozo W. Comparación de concentraciones de cadmio y plomo en lechuga de cultivos industriales, hidropónicos y orgánicos recolectada en supermercados, Guayaquil. Universidad de Guayaquil; 2020.
100. Cabascango L, Valdés L. Determinación microbiológica y de metales pesados en berro (Nasturtium officinale R. Br.) expendido en los diferentes mercados del Distrito Metropolitano de Quito. Universidad Politécnica Salesiana Sede Quito; 2016.
101. Estrella E, Yepez K, Atiaga O. Determinación de la concentración de arsénico total en cultivos de arroz en la provincia de El Oro y su relación con las propiedades físicas y químicas del suelo, agua y planta. Universidad de las Fuerzas Armadas; 2016.
102. Diaz Ubilla LE, Mendoza Hidalgo E, Bravo Bustamente M, Dominguez Vergara N. Determinación de Cadmio y Plomo en almendras de cacao (Theobroma cacao), proveniente de fincas de productores orgánicos del cantón Vinces. Espirales Rev Multidiscip Investig [Internet]. 2018;2(15). Available from: http://revistaespirales.com/index.php/es/article/view/213
103. Mite F, Carrillo M, Durango W. Avances del monitoreo de presencia de cadmio en almendras de cacao, suelo y agua en Ecuador. In: XII Congreso Ecuatoriano de la Ciencia del Suelo XII Congreso Ecuatoriano de la Ciencia del Suelo. 2010. p. 17–9.
104. Chávez E, He ZL, Stoffella PJ, Mylavarapu RS, Li YC, Moyano B, et al. Concentration of cadmium in cacao beans and its relationship with soil cadmium in southern Ecuador. Sci Total Environ. 2015;533:205–14.
105. Vega E. Relación de los niveles de cadmio en raiz, hojas, testa y almendra de cultivares de Theobroma cacao L. Universidad Técnica de Machala; 2019.
106. Condo J, Pernía B. Determinación de niveles de cadmio en granos de maíz (Zea mays L.) de la Costa y Sierra ecuatoriana. Rev Cient Cien Nat Ambien. 2018;12(2):In Press.
Received: 23 April 2022 / Accepted: 29 June 2022 / Published:15 August 2022
Citation: Benavides Á, Romero B, Pérez-Almeida I, Pernía B . Evaluation of the concentration of heavy metals in vegetables from Ecuador. Revis Bionatura 2022;7(3) 58. http://dx.doi.org/10.21931/RB/2022.07.03.58