2022.07.03.16
Files > Volume 7 > Vol 7 No 3 2022

Caffeine. A critical review of contemporary scientific literature.
Ostilio R. Portillo* 1 and Ana C. Arévalo 2
1 Faculty of Engineering, National Autonomous University of Honduras (UNAH), Tegucigalpa, Honduras.
2 Faculty of Chemistry & Pharmacy, National Autonomous University of Hondura (UNAH), Tegucigalpa, Honduras.
* Correspondence: [email protected]; Tel.: 504-9376-1660
Available from: http://dx.doi.org/10.21931/RB/2022.07.03.16
ABSTRACT
Caffeine is a secondary metabolite extensively studied for its stimulatory properties and presumed association with specific pathologies. This alkaloid is typically consumed through coffee, tea, and other plant products but is also an additive in many medications and confectionaries. Nonetheless, despite its worldwide consumption and acceptance, there is controversial evidence as to whether its effects on the central nervous system should be interpreted as stimulatory or as an addiction in which typical withdrawal effects are canceled out with its daily consumption. The following discussion is the product of an extensive review of current scientific literature, which aims to describe the most salient topics associated with caffeine's purpose in nature, biosynthesis, metabolism, physiological effects, toxicity, extraction, industrial use and current plant breeding approaches for the development of new caffeine deficient varieties as a more economical option to the industrially decaffeinated coffees currently available to caffeine intolerant consumers.
Keywords: biosynthesis, decaffeination, extraction, metabolism, physiological effects, plant breeding.
INTRODUCTION
Caffeine is a methylated xanthine (1,3,7-trimethylxanthine) 1-4 extracted from coffee beans for the first time by Friedlieb Ferdinand Runge in 1820 3-5, and which exact formula was proposed by Medicus in 1875.6,7 It is a natural alkaloid which name originated from the German and French words kaffee and coffee respectively, and it is present in more than 60 sub-tropical species 8,9 such as coffee (Coffea sp.), tea (Camellia sinensis L.),3,10-12 cocoa (Theobroma cacao), the guarana plant (Paullinia cupana),10-12 the mate grass (Ilex paraguariensis St. Hil.) 11 among others.
Caffeine is a white, odorless, water-soluble compound 4,12,13 and its solubility increases as the water temperature increases.14 For example, the solubility of caffeine is 0.6% at 0 °C (32 °F), 13% at 25 °C (77 °F) & 66.7% at 100 °C (212 °F).
Depending on the concentration, it contributes to the bitter taste of the drink 4,15-17 and is held responsible for no more than 10 to 20% of the bitterness perceived in the coffee drink.6,18-21 However, according to some authors, their contribution to the drink's bitter taste is so low that it plays a minor role in the definition of flavor.22
This alkaloid is thermostable, so it is not degraded while roasting coffee beans.2,22-24 However, the small losses that occur are generally attributed to sublimation due to the high temperatures during roasting 4,6,22,25; When this occurs, the relative increases are recorded in its concentration (≤ 10%) are attributed to the loss of other compounds during the grain roasting.6
Caffeine in the form of a drink such as tea, coffee, and cola, among others, is one of the most socially accepted natural stimulants which acts on the central nervous system (CNS) as an antagonist of adenosine receptors, being the most consumed and studied psychoactive substance in history.18,26
Biosynthesis and purpose
Although caffeine was isolated from coffee beans for the first time in 1820 3 it was not until 1895 that the first report of its artificial synthesis was recorded 27,28 from dimethylurea, and most of the methods known to date were patented between 1940 and 1960. Artificial synthesis of caffeine is a cheap process carried out from theophylline, uric acid or uracil.
In the plant, the biosynthetic pathway of the purine nucleus alkaloids, such as caffeine, starts from the inosyl monophosphate, which generates the xanthosine 5'-monophosphate, and when the phosphate group is removed xanthosine is produced from which a four-stage route initiates in the following sequence: xanthosine (I) → 7-methylxanthosine (II) → 7-methylxanthine (III) → theobromine (IV) → caffeine.
The first, third and fourth stages involve three methylations catalyzed by three N-methyltransferases at positions 7-N, 3-N, and 1-N 22,29-31 and S-adenosyl-L-methionine (SAM) being responsible for the donation of methyl groups (-CH3) during the methylation reactions (Figure 1).22,31
The evidence suggests that caffeine is synthesized in planta in the young leaves and in the pericarp from where it is translocated and accumulated in the endosperm of the seeds of C. arabica and C. canephora 6,8,10,22,30,32; However, its production occurs only during the early stages of fruit development 19,31 which was evidenced by the results obtained by Perrois et al.30 who quantified the alkaloid concentrations during different stages of bean development in different genotypes of both species.

Steps II, III and IV represent methylations. Step II represents the separation of a ribose. SAM = S-adenosyl-L-methionine & SAH = S-adenosyl-homocysteine.
Figure 1. Caffeine's biosynthesis. Steps II, III and IV represent methylations. Step II represents the separation of a ribose. SAM = S-adenosyl-L-methionine & SAH = S-adenosyl-homocysteine.
The results suggest a more remarkable caffeine synthesis in both species' early stages of bean development with a more significant accumulation of the alkaloid in Robusta coffee varieties (Figure 2). Perrois et al. 30, the lower accumulation of the alkaloid in Arabica coffee varieties have its origin in its genomic constitution.
In addition, caffeine as a secondary metabolite is not only present in the leaves (~ 0.8% based on dried matter),10,33-35 but in the fruit pericarp also (e.g., exocarp, mesocarp, and endocarp) 10,19 and in the coffee flowers where it reaches the highest concentrations compared to other structures of the plant.35 Benatti et al. 36 reported that caffeine is present in the flowers of C. arabica during anthesis (period during which the flower remains open and functional), with an average concentration of 0.58 mg g-1 in the petals and gynoecium and 1.36 mg g-1 in the stamens.
Sequential molecular analyses of deoxyribonucleic acid (DNA) suggest that C. arabica is the product of the natural fusion of two chromosomal sets of two diploid species of the genus Coffea, presumably C. eugenioides (♀) and one species of the canephoride group probably C. canephora (♂) 22,37-41 with a single polyploidization event after the merger 42 or it probably arose through the fusion of two non-reduced gametes of the above-mentioned species.43
For this reason, C. arabica is classified as a segmental allotetraploid (CaCaEaEa).39,40,44 The lower caffeine accumulation in Arabica coffee varieties could be attributed to a significant reduction in the transcriptional activity of enzymes responsible for the alkaloid synthesis in C. eugenioides, which in turn would reduce the transcriptional activity of enzymes derived from homeologs genes of the Robusta's sub-genome.30,31
Its in planta function has been debated for years, but two hypotheses prevail the protection of leaves and fruits from predators, coupled with an allelopathic effect that disturbs the germination of adjacent seeds and development of newly germinated neighboring plants.10,31,34,45,46 Germination tests conducted by Ransom 47 confirm that caffeine can delay and even prevent seed germination and seedling development of multiple horticultural species even at low concentrations (1%).
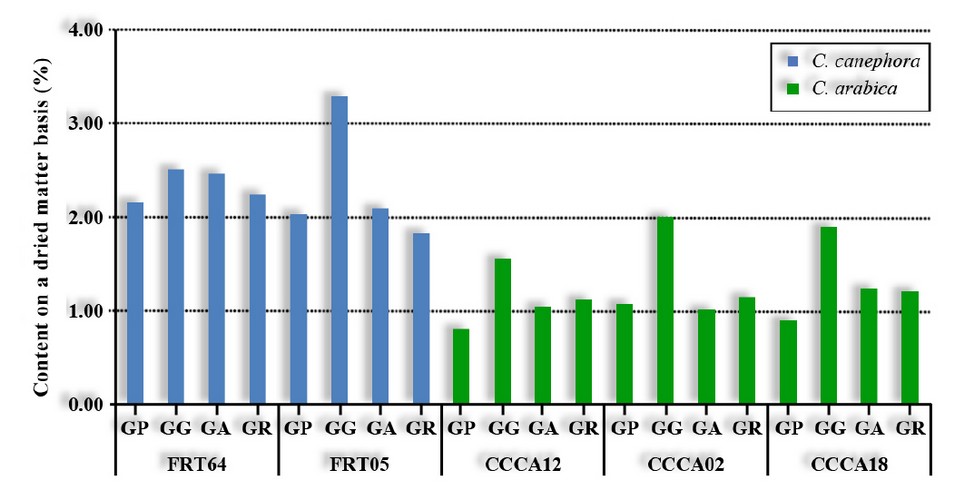
Figure 2. Caffeine content at different stages of bean development in two varieties of robust coffee & three varieties of Arabica coffee. Genotypes of C. canephora Robusta variety: FRT64 & FRT05. Genotypes of C. arabica Caturra variety: CCCA12, CCCA02 & CCCA18. Stages of grain development: SG, small grain; LG, large grain; YG, yellow grain and RG, red grain. Adapted from Perrois et al. 30
The caffeine content in coffee
The species of the genus Coffea display a wide variation in their caffeine concentration on a dry basis, ranging from 0.6-1.4% in C. arabica and 1.8-3.5% in C. canephora 2,4,21,23,48 to a very low or complete absence as in the case of C. salvatrix and C. pseudozanguebariae respectively.30,49,50
Due to its higher caffeine content, coffee prepared with C. canephora can have concentrations ranging between 56-203 mg 100 ml-1 of infusion, while those prepared with C. arabica have levels ranging between 36-112 mg 100 ml-1 of infusion.51
The observed variations in the coffee drink can be attributed to: the cultivated species or variety (Table 1) since it is an inherited trait,10,40 the environment (e.g., soils, planting height above sea level and the degree of light exposure); the chosen roast level, averaged particle size after grinding, the method of drink preparation, the cup size,52,53 the proportion of water and roasted coffee and blends made before or after roasting (% of Arabica vs % of Robusta).4,11
Based on the evidence, it can be deduced that the bean's caffeine content is conditioned by the degree of light exposure. Interestingly, coffee plants grown under shade tend to have a more significant alkaloid accumulation than those sown directly under the sun, regardless of the species involved.
For example, a study conducted on C. canephora plants grown under complete darkness revealed the alkaloid accumulation in plant tissues plummeted; Additionally, a reduction in the transcriptional activity of genes involved in its biosynthetic pathway (ie, CcDXMT, CcXMT, & CcMXMT) was also recorded; However, this trend was reversed after 6 to 12 hours of light exposure 31 which suggests that although light is necessary to induce caffeine production the amount of required exposure is low.

* Percent contents based on dried matter.
Reproduced with permission of Oestreich-Janzen 22.
Table 1. Caffeine Content by Species and Coffee Varieties
On average, the raw coffee bean contains ~ 1% of caffeine 27; However, one cup of coffee contains ≤ 100 mg of caffeine; and the maximum recommended intake should not exceed 400 mg day-1 (~ 5.7 mg kg-1 live weight),4,11,54-57 the equivalent of four cups of coffee.11,56-58 Fortunately, the estimated average daily consumption for regular coffee consumers in the USA ranges between 186 to 200 mg.59,60
Additionally, according to the Specialty Coffee Association of America (SCAA), the average consumption of coffee in the USA is 3.1 cups per person per day.61,62 In contrast, according to Greenberg et al.1 "The Canadian Government's recommendations through its guidelines for healthy eating is to limit the intake of caffeine between 400 to 450 mg day-1 which translates into approximately three 8-ounce cups of coffee per day" for adults. At the same time, for children and adolescents, the recommended doses are 2.5 mg per kg of live weight.11
Although caffeine is not considered an essential nutrient in the human diet, it is still used in formulating various products. Table 2 shows a series of popular drinks with their respective caffeine contents expressed as average values and ranges.

Table 2. Caffeine Concentration in Selected Common.
Caffeine determination
Caffeine is detected in food samples with trigonelline and nicotinic acid depending on the analytical method used.50 Its determination in food samples is carried out through different analytical methods that allow its identification and quantification in complex matrices.
Among the commonly used analytical methods, we can mention gas chromatography,17,63 thin-layer chromatography,17 the Kjeldahl method based on the quantification of nitrogen (N2) present in the caffeine molecule,63 high-performance liquid chromatography (HPLC), ultraviolet light spectrophotometry which is performed at a wavelength of 280 nm,12,17,22,63 near red light spectroscopy (NIR), capillary electrophoresis,12 mass spectroscopy 17 among others.
Unfortunately, implementing these analytical methods requires very sophisticated and expensive equipment operated by highly qualified technicians, which increases laboratory analysis costs. On the other hand, these analytical methods (except for HPLC) require a series of previous procedures (aka sample preparation) to remove, as much as possible, unwanted analytes that interfere with the analysis, turning the caffeine determination into a laborious, expensive and impractical task for repetitive purposes.
To remove unwanted analytes, Molecular exclusion chromatography, also known as Size Exclusion Chromatography (SEC), Solid Phase Extraction (SPE) or a combination of both, is usually applied as part of the same protocol, which in turn increases the cost and time required for the elimination of interfering compounds.
The SEC also known as gel filtration chromatography, is based on a mechanically resistant stationary phase (e.g., agarose, Sephadex, silicone gel, polyacrylamide), which because of its homogeneous porosity, acts as a filter or sieve. On the other hand, SEC also has a mobile or liquid phase (e.g., acetone, EtOAc, acetonitrile, EtOAc-n-hexane, MeOH, n-hexane), which facilitates its movement through the gel.
SEC allows compounds separation based on their molecular weight, depending on their size. When moving through the stationary phase, the eluted molecules can be temporarily retained in the gel pores when they are very small, thus separated from the other compounds in a heterogeneous matrix. In contrast, intermediate weight molecules are partially retained, which slows their displacement through the stationary phase, thus separating them from larger molecules that, due to their size, aren't retained and move more rapidly (Figure 3).

Figure 3. Schematic description of size exclusion chromatography (SEC).
The speed of molecules displacement through the gel affects the results of the chromatographic elution profile since, graphically speaking, the first peaks correspond to the larger molecules, followed by those of intermediate size and finally, the peaks corresponding to the minor compounds appear.
Finally, because the mobile phase is usually an organic solvent, the first compounds to pass through the stationary phase are the high molecular weight lipids in the matrix.
In contrast, SPE is the most common cleaning technique and is similar to SEC because it also has a mobile and stationary phase that can be manufactured based on activated silica, polymers, activated carbon or alumina. However, the SPE is generally used to purify and concentrate the analytes of interest by removing impurities that act as interfering compounds in chromatographic analyses.
This technique is based on the fractionation of the constituents of a heterogeneous matrix based on their affinity for the stationary phase. First, the heterogeneous matrix is dissolved in the mobile phase (e.g., MeOH, acetonitrile, tetrahydrofuran, etc.) and then filtered or centrifuged to remove any unwanted particles. Then, the recovered liquid is circulated through the separation column (stationary phase).
If the compounds of interest lack affinity for the stationary phase (ie, equal charges), then they circulate freely through it, leaving behind unwanted impurities, thus allowing the purification and concentration of the analytes of interest. For example, octadecyl, more commonly known as C18 is generally used as a constituent of the separation column for the retention of non-polar compounds and lipids, which are adhered to thereto through hydrophobic interactions.
In contrast, if the compounds of interest show an affinity for the stationary phase (ie, opposite charges), then they're retained therein, allowing the free circulation of later discarded impurities. In the process, it is possible to clean and concentrate the analytes of interest recovered from the stationary phase when flushed out with another solvent (Figure 4).

Figure 4. Schematic description of solid phase extraction (SPE).
However, when both parties exhibit affinity for the stationary phase, a solvent strong enough to remove the interfering compounds should be used during the rinse but not too strong to remove the analytes of interest. Generally, this is achieved with the same eluent used in the preparation of the mobile phase but attenuated, that is, at a lower concentration.
After sample preparation, identifying the compounds present in the heterogeneous matrix results from a correlation or comparison between the retention time (Rt) of unknown molecules with the Rt of known references.
According to Pizzariello et al. 17 a simple and rapid technique to clean aqueous coffee samples before caffeine's determination consists of the flocculation of the interfering compounds. By adding a coagulating agent, aggregates can be formed, which are separated from the aqueous phase via precipitation, filtration or decantation, thus eliminating from the matrix all the compounds bound to the coagulating agent which would affect the chromatograph resolution. Otherwise, the interfering analytes are plotted in the chromatograph as peaks of unknown nature (artifacts).
Decaffeinated coffees
At present, the only caffeine-free coffee options available on the market are those that have been decaffeinated through industrial processes. In 1905 Ludwig Roselius registered the first patent for decaffeination 22,27, and the first commercial decaffeination plant owned by Kaffee HAG began operations in Germany in 1906. But it was until 1908 that the first successful decaffeination process was patented on behalf of Meier, Roselius, and Wimmer.4
Nowadays, the production of decaffeinated coffees represents between 10 to 15% of coffee sales in the world 18,19,64-66 with the USA being the largest consumer; and more specifically, it is consumed by people who due to a health condition (eg, cardiovascular disease, insomnia, etc.) have to avoid consuming drinks that contain caffeine.4,40,66,67
However, caffeine is not the only metabolite blamed for the discomforts experienced by such consumers. Other compounds in the coffee drink that can cause irritation to the digestive tract (stomach mucosa) are pyrogallol, catechol, N-alkanoylhydroxytryptamide, N-methylpyridinium, & chlorogenic acids.68
It should be noted that decaffeinated powdered coffee is not completely free of the alkaloid because on average, it contains 0.05% of caffeine 2,69; However, in North America (ie, the USA and Canada) for a coffee to be considered decaffeinated, 97% of the alkaloid must be removed 4,22 which, for a 5 oz cup, is equivalent to a concentration not higher at 5 mg.61 In contrast, according to European legislation, soluble decaffeinated coffees should not exceed 0.3% caffeine content.4,22,70
The decaffeination process is performed before roasting,18 it is expensive and affects the product's organoleptic properties 71 due to a greater or lesser degree of leaching of secondary metabolites depending on the applied decaffeination method.4 In addition, changes in the structure, size, and appearance of the grain, loss of mass and residual accumulation of organic solvents are also recorded.4
For example, there is evidence that raw decaffeinated coffee beans contain approximately 1.2 times more chloro-genic acids (eg, monocaffeoyl-quinic, mono-feruoylquinic, and dicaffeoylquinic acids) than non-decaffeinated raw beans.66 The increase in the concentration of chlorogenic acids can be attributed to the removal of other secondary metabolites such as caffeine.
There are several methods for decaffeination of coffee, such as the Swiss water-based process, solvents, and supercritical fluid extraction.
The Swiss water-based process
One of the first decaffeination patents associated with this method was registered in favor of Francis R. Eden in 1917.67 In general, the process takes approximately 8 h 4, and it proceeds as follows:
a) Fresh coffee beans are immersed in hot water (93-104 °C) to solubilize the caffeine. Then, the extract, rich in caffeine and other substances, is passed through an activated carbon filter whose porosity retains caffeine molecules but allows the free passage of other smaller molecules 4 whose removal affects the organoleptic properties of the grain of coffee.
In this way, the caffeine content of the beans is reduced, but also an essential amount of substances that affect their flavor and aroma (e.g., sugars, lipids and phenolic compounds).4
b) The recovered caffeine-free aqueous extract is used to repeat the operation with a new batch of grain.4 Since the operation is repeated using the aqueous extract rich in solubilized substances, then, in theory, the grains lose only the caffeine, which is removed via filtration.
c) A variation to this process involves the reincorporation of the recovered aqueous concentrate (caffeine-free) into the decaffeinated grain batch before drying it (Figure 5).4 In theory, the metabolites that shouldn't be extracted during the decaffeination process are reincorporated into the decaffeinated grains.
However, despite the effort to reinstate secondary metabolites solubilized by water, there are reports of chlorogenic acid losses (~ 20%) due to the decaffeination process with water.18

Figure 5. Water-based caffeine extraction scheme.
The use of solvents
One of the first patents associated with this decaffeination method was registered in favor of Friedrich Meyer et al. in 1908, but benzene was used in his method as an organic solvent which eventually ceased to be used due to its toxicity.67
Presently, decaffeination through organic solvents is the most common and cost-efficient process used by industry. Coffee beans are incubated in a steam bath to open their pores and increase their moisture by 12 to 40%,4,22,70 increase the solubility of caffeine and dissolve caffeine-based complexes with other molecules. Then, they are sprayed with dichloromethane, dichloroethylene, hexane, methylene chloride, diisopropyl ether or ethyl acetate (EtOAc) to solubilize and remove caffeine molecules.4,18,22,67,70 The process takes approximately 10 h to remove the organic solvent from the coffee beans.
Subsequently, the grains are dried up between 10 to 12% of humidity,4 the solvent mixed with the caffeine is processed to separate them and recover a pure extract of caffeine which is used in other industrial applications such as the production of medicines and carbonated drinks.4,70 In general, the solvent is separated from caffeine via distillation, thus recovering a heterogeneous solid commonly called "coffee wax" composed of 60% caffeine and 40% of other substances, mainly lipids (triglycerides) (Figure 6).4 In the end, caffeine levels in the beans are reduced between 0.02 to 0.3% on a dried weight basis.65
Trichloroethylene was used as a solvent until 1977, when it was removed from the process because it was considered a carcinogen. Nowadays, methylene chloride is used as this is a more specific organic solvent than water, but still, the process removes other compounds (eg, chlorogenic acids), thus affecting the coffee's organoleptic properties. Farah et al.72 reported the analysis of decaffeinated and roasted coffee samples of C. arabica showed a 10% loss of chlorogenic acids compared to non-decaffeinated and roasted samples under the same conditions.

Figure 6. Scheme of caffeine extraction based on organic solvents.
On the other hand, the Food and Drug Administration (FDA) and the European Union allow, in roasted coffee beans, a maximum dichloromethane concentration of 10 4,70 and 3 mg kg-1,, respectively; However, the levels reported by industry are 100 times lower because its boiling point is 40 °C (104 °F) and during roasting the grain is exposed to much higher temperatures (210-240 °C) with that any residue thereof that could have been left in the grain after decaffeination is volatilized.18
Additionally, the FDA allows a maximum of 10 ppm as a residual effect of methylene chloride in decaffeinated coffee samples. However, EtOAc is a non-chlorinated but flammable solvent that was introduced as an alternative to chlorinated compounds because it is considered a natural compound since it is present in small quantities and in certain ripe fruits.4
Currently, there are no maximum permissible limits established for EtOAc in decaffeinated coffee 4; However, EtOAc has certain disadvantages, such as a 5 to 6% solid leaching.
Supercritical fluid extraction (SFE)
The process is based on the patent registered in 1970 by Studiengesellschaft Kohle of Mulheim, Germany, which demonstrated that supercritical carbon dioxide (CO2) is a more selective solvent for caffeine. The SFE is the most expensive process used by industry. However, it helps to remove metabolites from the endosperm through supercritical fluids using different solvents that act selectively and has the advantage of a shallow residual effect, thus eliminating the problem associated with other decaffeination methods.
In addition, since the process is applied to raw grains, the compounds responsible for the characteristic smell and taste of roasted coffee are not removed, so the sensory qualities of roasted and ground coffee, as well as those of the beverage, are not affected.
CO2 is the solvent of choice for the extraction of caffeine from coffee beans because, however, it doesn't dissolve it as efficiently as other solvents 4: it is inert, it isn't corrosive, it isn't combustible, it isn't explosive, it is abundant and cheap, it isn't toxic, it is a harmless ingredient in drinks and food, it is easily separated from the metabolite of interest after extraction, it has a low molecular weight, a low surface tension and quickly diffuses into the sample. In addition, it presents a low critical temperature (Tc: 31-31.8 °C) and critical pressure (Pc: 7.39 MPa).4,73,74
SFE can be used in industrial processes such as decaffeination of caffeine-containing products (eg, coffee, tea, cocoa, mate grass, etc.) or for the recovery of specific secondary metabolites such as caffeine, lipids, and N-alkanoyl-5-hydroxytryptamides (C5HTs).67,75
Caffeine solubility increases with increasing pressure and temperature, which is why the process is conducted at temperatures greater than 31 °C (87.8 °F) and pressures above 1000 lb per square inch.75
Raw coffee beans are soaked in water until they reach a 45% humidity to then be placed in a sealed container. Then CO2 is injected into it at a pressure of approximately 200 atm. CO2 is specific for caffeine, so it is removed, leaving behind all other secondary metabolites, thus increasing their relative concentration.
Subsequently, the mixture of CO2 and caffeine is transferred to another chamber where the pressure is released, and the CO2 returns to its gaseous state, while the caffeine and precipitated lipids are passed through an activated carbon filter. While the CO2 is reused in the decaffeination of a new batch of grain; the isolated caffeine is used in the manufacture of carbonated beverages and medications (Figure 7).4
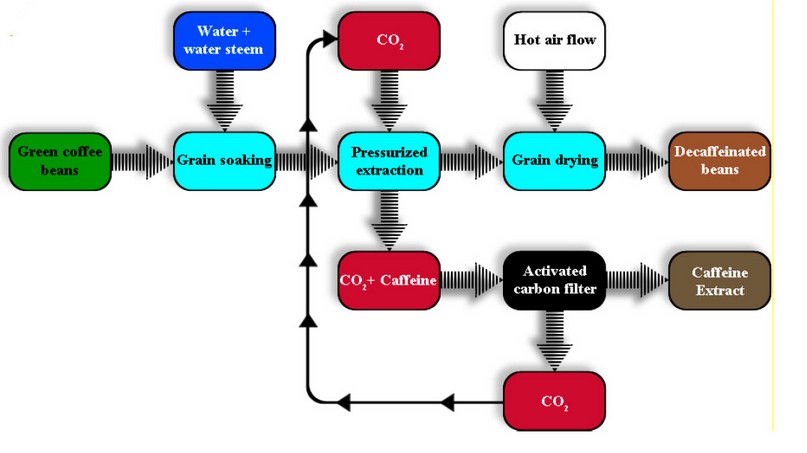
Figure 7. Scheme of caffeine extraction through supercritical fluids.
Decaffeinated coffee beans have a drastic reduction in caffeine levels (~ 0.02%), are more susceptible to fungal attack 76 and display a brown or yellowish color extending from the surface across its entire transverse section.4,77 Such pigmentation is attributed to high molecular weight melanoidins produced by high temperatures during the Maillard reaction.77
Melanoidins are brown water-soluble polymers that occur when the carbonyl groups (C=O) of reducing sugars condense with the amino groups of polypeptides (e.g., enzymes and proteins).77
Breeding of coffee varieties
A cheaper and simpler alternative that doesn't involve the use of organic solvents, which leach other secondary metabolites, thus altering the organoleptic properties of coffee beans, whose residuality is not well seen by consumers, is the development of caffeine-deficient varieties through plant breeding.
Caffeine content is a highly heritable polygenic and quantitative characteristic (strict inheritance = 0.80) in the case of intra or inter-specific crosses,42,78, so the development of varieties with low levels of caffeine is feasible through crosses and selections. Selection alternatives include, but are not limited to:
a) The development of caffeine-deficient isogenic lines through controlled introgression between the most productive varieties of C. arabica and C. pseudozanguebariae or C. charreriana, which don't synthesize caffeine.10 The absence of caffeine in some germplasms is caused by the expression of a recessive gene (caf1) when it is present in a homozygous state.42
b) Germplasm improvement based on spontaneous mutations. The cross between highly productive varieties of C. arabica and the Ethiopian AC1 mutant displays an average caffeine accumulation of 0.76 mg g-1.36 The low caffeine accumulation is attributed to a reduction in the methyltransferase activity responsible for theobromine methylation, a caffeine's precursor, causing a systematic theobromine accumulation in plant tissues (Figure 1).36
c) Induced-mutation breeding. One case that exemplifies this alternative is the chemically induced mutant called Decaffito which originated when a Red Catuaí seed sample was exposed to sodium azide for 48 h resulting in the interruption of theobromine methylation, which causes the latter one to be systematically accumulated, thus interrupting the caffeine biosynthesis.35
This mutation follows a Mendelian segregation pattern (3:1), which means that its expression is recessive and is characterized by presenting smaller flowers with the capacity to produce viable pollen, early anthesis and a clear tendency for cross-pollination.35
Metabolism
Caffeine is rapidly absorbed after ingestion 22,55,79, and due to its hydrophobic nature, it crosses the stomach and small intestine cell membranes through passive diffusion; Once absorbed, it passes into the plasma blood where it is detected approximately five minutes after intake 80 and then is distributed to all body tissues.2,52,81
It should be noted that when caffeine is ingested in isolation, through drinks that contain it, its rapid absorption and circulation in the bloodstream can be observed; however, when its intake is produced together with food, its absorption slows down, so the concentration of the alkaloid in the blood plasma is reduced.60
Although the caffeine's half-life (T½), in adults is approximately 5-6 hours 2,11,80 it can be extended due to a slower metabolism, as in the case of pregnant women (T½ = 11.5-18 h) 2,11,52,53 whose caffeine metabolism slows from the fourth month until delivery for reasons still unknown.28,53,70
In addition, the simultaneous consumption of nicotine and caffeine causes an increase in T½ of the latter due to a slowdown in its metabolism attributed to the effect of nicotine on the metabolic functions of the CYP1A2 enzyme.82 Similarly, certain antidepressants such as Fluvoxamine have the ability to inhibit CYP1A2 enzyme functions, thereby altering the metabolism of caffeine and indirectly increasing its T½.82
On the other hand, although there is no evidence to show beyond any doubt that excessive caffeine consumption interferes with fertilization,11 there are records that suggest an excessive intake has been correlated with spontaneous abortions 83,84 and the consumption of more than 600 mg/day during pregnancy is capable of inducing stunted growth and weight gain of the fetus concerning its expected chronological development during pregnancy.11,83-85
In addition, it isn't possible to rule out the possibility that caffeine consumption during pregnancy impacts the fetus's neural development with subsequent repercussions on the individual's behavior during his life.5 For all the above, the daily consumption of caffeine for pregnant, lactating women and for those who think about becoming pregnant shouldn't exceed between 200 to 300 mg.11,56,83,86,87
Women who take birth control pills, children (infants T½ = 80 h & 6 months of age T½ = 2.6 h), smokers and people with liver disease also metabolize caffeine slowly (T½ = 50-160 h) 2,28,52,60,84,86 which makes them more susceptible to its effects. For this reason, it is recommended that the daily caffeine consumption for children shouldn't exceed 2.5 mg kg-1 of live weight.83
Caffeine reaches its maximum concentration (Tmax), in blood plasma and breast milk, one hour after ingestion 2,11,22,81 and then it is inefficiently eliminated through the kidneys as it is rapidly reabsorbed in the kidney's tubules.13,79
Ingested caffeine is 95% metabolized in the liver by the enzyme cytochrome P450 oxidase (Isoforms: CYP1A1 & CYP1A2 AA) 2,53,81,86,87 in three dimethylxanthines with pharmacological action: (a) through a 3-N-demethylation ~ 70% is converted to paraxanthine (1,7-dimethylxanthine), (b) 25% is transformed into a mixture of theophylline (1,3-dimethylxanthine) and theobromine (3,7-dimethylxanthine) through of a 1-N-demethylation and a 7-N-demethylation respectively and finally (c) about 5% is oxidized without demethylation to form uracil and uric acid compounds (1,3,7-trimethyluric acid) (Figure 8).2,79,81
Subsequently, these metabolic derivatives are demethylated again by turning them into the corresponding monomethylxanthines before being oxidized in uric methyl acids and other derivatives. However, although most of the ingested caffeine is metabolized, a small percentage (3-6%) is excreted through the urine without changes 11,13,22 together with its metabolic derivatives.22
In addition, caffeine influences hepatic lipid metabolism and has a proven antioxidant activity 88-90 which is similar to that of glutathione and more significant than that of ascorbic acid.79 In addition, caffeine's antioxidant capacity was evaluated in a study in which oxidative stress was induced, in rodent liver cells, through the exogenous application of hydroxide radicals (OH-), peroxide radicals and oxygen molecules (O2).
The results indicated that caffeine, at low concentrations, could avoid lipid peroxidation caused by free radicals; However, its antioxidant effect was more significant when O2 molecules were applied and lower when hydroxides were used.79
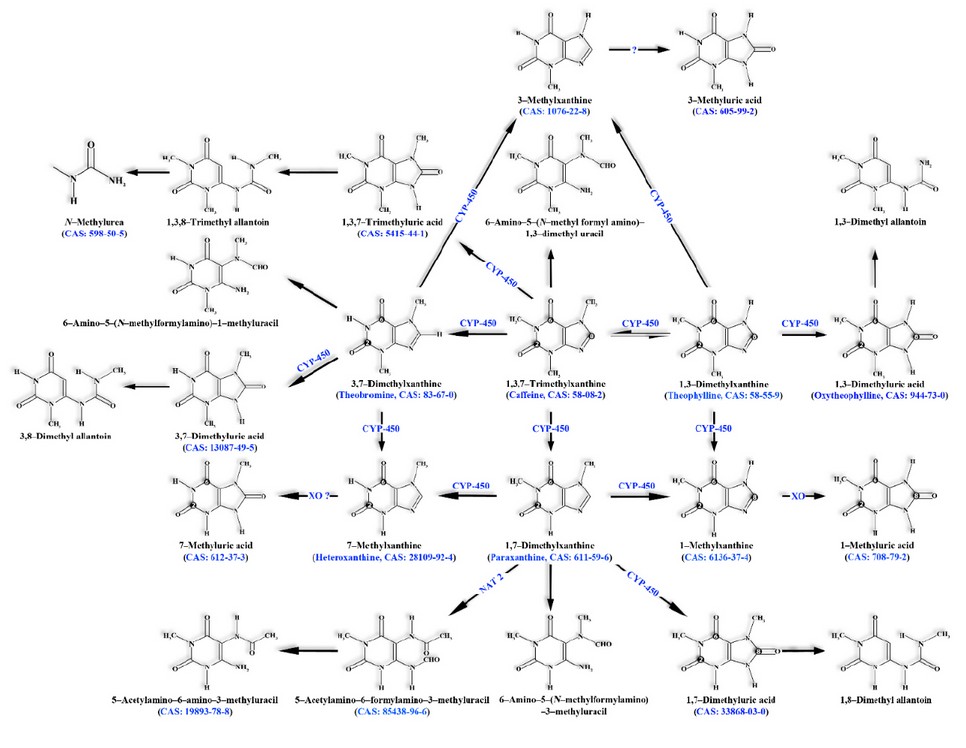
CYP: Cytochrome P450 | NAT 2: N-acetyltransferase 2 | XO: Xanthine oxidoreductase.
XO ?: unknown Xanthine oxidoreductase. Adapted from Ludwig et al. 2
Figure 8. Human hepatic caffeine metabolism.
Subsequently, these metabolic derivatives are demethylated again by turning them into the corresponding monomethylxanthines before being oxidized in uric methyl acids and other derivatives. However, although most of the ingested caffeine is metabolized, a small percentage (3-6%) is excreted through the urine without changes 11,13,22 together with its metabolic derivatives.22
In addition, caffeine influences hepatic lipid metabolism and has a proven antioxidant activity 88-90 which is similar to that of glutathione and more significant than that of ascorbic acid.79 In addition, caffeine's antioxidant capacity was evaluated in a study in which oxidative stress was induced, in rodent liver cells, through the exogenous application of hydroxide radicals (OH-), peroxide radicals and oxygen molecules (O2).
Physiological effects
Caffeine exerts a psychoactive action that stimulates the central nervous system (CNS) 19,91-93 because its molecular structure is very similar to that of adenosine; an endogenous neurotransmitter with an inhibitory effect capable of regulating sleep and arousal 7,83 and therefore acts as an antagonist of the latter one 1,2,92,94 that is to say that it competes with adenosine by coupling with its receptors (ie, A1, A2A, A2B, & A3).
The activation of the A1 and A2A receptors specifically affects the CNS because type A1 receptors are distributed throughout the brain mass with a greater concentration in the hippocampus and hypothalamus as well as in the cerebral cortex and cerebellum 2,5,11,95 while type A2A receptors are concentrated in brain regions rich in dopamine such as the striatum, the globus pallidus and the subthalamic nucleus 2,5,60,96 where, when activated, they promote its synthesis.79
However, type A2A receptors can also be found in tissues not associated with the CNS (e.g., the cardiovascular system), where when activated, they promote tissue repair, wound healing, collagen production as well as reducing inflammation and protecting the liver from acute inflammations.28,79
Adenosine inhibits the spontaneous release of acetylcholine, norepinephrine (the hypothalamus' main neurotransmitter), dopamine, serotonin, and γ-aminobutyric acid.97 However, when competing with adenosine for the same receptors (A2A), caffeine causes the opposite effect, an increase in dopamine levels which in turn causes stimulation of the CNS.11,87 Similarly, caffeine also increases the blood plasma concentration of serotonin, acetylcholine, γ-aminobutyric acid and glutamate.87
Caffeine effects aren't limited to the CNS because it also affects the sympathetic nervous system when it interacts with its primary neurotransmitters (i.e., adrenaline and norepinephrine).2,81,98
Unfortunately, the evidence indicates that caffeine at low concentrations (10 and 20 mg kg-1) worsens cases of acute liver inflammation; Conversely, at high concentrations (100 mg kg-1) completely blocks inflammatory responses through another mechanism non-related to the A2A receptors.79
The action of adenosine in both receptors has the opposite physiological effects. When adenosine is coupled to the A1 receptors, it induces vasoconstriction that affects the renal arterioles, which in turn translates into a reduction in blood flow and a decrease in the filtration rate.81 In contrast, when coupled to A2A receptors, it causes vasodilation of the renal arterioles; However, the effect of the first cancels the effect of the second, so the result is vasoconstriction.
However, when caffeine successfully competes with adenosine for A1 receptors, the result is vasodilation that causes a greater rate of filtration, urine output, increased blood flow and reduced blood pressure.81 This result is to be expected since one property that characterizes xanthines is their vasodilator effect, which is why they are used as bronchodilators.3,26,85
In contrast, evidence indicates that caffeine acts as a vasoconstrictor at a brain mass level thus, it causes a decrease in blood flow in the brain.82 If we consider that many headaches are associated with increased blood circulation in the brain mass, then it can be understood why several medications formulated for migraines contain caffeine.28,82
Caffeine also can improve attention (the ability to concentrate on one task while ignoring less relevant ones), surveillance (the ability to maintain performance in a task for extended periods), reflexes and information processing speed 9,11,65 at a dose ≤ 100 mg 28 within the parameters established by the law of Yerkes-Dodson.60 According to Yerkes-Dodson law, there is a relationship between the degree of stimulation and the performance of an individual in such a way that an adequately stimulated person will have a high degree of performance and vice versa.
For example, administering caffeine to a fatigued person will cause performance improvement. However, when a state of hyperstimulation is reached, performance decreases. In such a way that when administering caffeine to a previously stimulated individual, the obtained effect is contrary to the expected.
In addition, caffeine also improves long-term memory and symptoms associated with Parkinson's disease (e.g., tremors) through dopamine secretion.2 On the other hand, the evidence suggests that caffeine confers a certain degree of protection against the development of skin cancers induced by ultraviolet light.9,27
Finally, there is a significant correlation between the antioxidant capacity of coffee and caffeine, which suggests that the latter is an antioxidant in itself, contributing to reducing the concentration of free radicals (eg, NO+, NO-, ONOO-, H2O2, O2-, NO, & OH-) in the body.50
Caffeine and physical fatigue
Caffeine has an ergogenic effect that reduces the feeling of fatigue when performing exercises or physical work 1,2,19 which is why athletes from multiple disciplines consume it legally as a supplement.82 In addition, the ergogenic effect didn't go unnoticed by many American companies which, during World War II, offered to their workers coffee for free since an increase in productivity was demonstrated and by the end of 1952 80% of the companies had instituted what is now known as the coffee break.99
However, the evidence indicates that caffeine metabolism varies significantly between people in such a way that the time required to reach the maximum blood concentration (Tmax) can range between 0.5 to 3 h.60 Curiously, the evidence suggests that the alkaloid ergogenic effects are not linked to its Tmax, that is, that such effects are observed under a wide range of concentrations in different individuals as long as the concentration in the blood plasma doesn't plummet below 15 to 20 µM.60
Due to its proven ergogenic effect and rapid absorption through the oral mucosa, the Department of Defense of the United States of America provides its members with chewing gum whose formulation contains caffeine and adds it to specific military rations.60 Consequently, it could be speculated that workers who perform jobs that require high physical performance could benefit from its stimulating effects, as in the case of athletes or those who need to remain alert for extended periods, as in the case of drivers with nighttime shifts, aviation pilots, hospitals' medical staff among others.
Unfortunately, due to its ergogenic effect, the International Olympic Committee introduced an anti-doping program in 1984 through which an athlete can be disqualified if their caffeine levels reach 12 µg ml-1 of blood plasma which is tantamount to consuming 9 mg of caffeine per kilogram of live weight one hour before the test.11,60
However, in 2004 the World Anti-Doping Agency removed caffeine from the list of prohibited substances due to the difficulty of determining whether the such concentration is due to differences in people's metabolic efficiency or to social or intentional consumption before a sports competition.11,60
Caffeine and gastric acidity
Multiple reports confirm a connection between coffee consumption and an increase in gastric acidity caused by an increase in gastric acid (HCl) secretion 13,67,100, which in some susceptible individuals or those who suffer from a Helicobacter pylori infection can cause acute stomach acid and gastroesophageal reflux after drinking coffee.67
However, experiences reported by Pehl et al. 101 suggest the intake of decaffeinated coffees is a viable option for patients suffering from gastric acid and gastroesophageal reflux. A study conducted to evaluate the effect of caffeine on gastric acid secretion was attended by 10 healthy volunteers who were supplied with 50 ml of a control solution (10 g of glucose in 100 ml of water), coffee drink (240 mg of caffeine in 100 ml) and decaffeinated coffee drink (0.1 mg of caffeine in 100 ml).
The results indicate that standard coffee increased gastrin secretion 2.3 three times more compared to the secretion observed in controls, while decaffeinated coffee increased gastrin secretion only 1.7 times.67 Gastrin is a linear peptide secreted by the stomach parietal cells (G cells) and has a hormonal function because it regulates the secretion of HCl in addition to stimulating the growth of the stomach mucosa in regions responsible for the secretion of HCl.
The evidence shows a significant reduction in the degree of esophageal acidity and a reduction in the amount of gastroesophageal reflux due to the ingestion of decaffeinated coffee produced through CO2-based -based supercritical extraction.101
Caffeine and osteoporosis
The claims that caffeine is positively correlated with osteoporosis, in men and especially in postmenopausal women, expressed by a reduction in bone density and an increase in the number of fractures, are based on inconclusive experiences, so the subject continues to be a scientific debate.
Osteoporosis is a condition in which the apatite crystals break off with the subsequent degradation of bone, and although the process is also called decalcification, the truth is that more than simply calcium is lost in the process.
In general, the data suggest that an increase in caffeine intake reduces the efficiency of calcium absorption in the digestive tract,5,83 reduces bone density thereby increasing the risk of fractures 5 while calcium and magnesium excretion increases through the urine 1,18,102 especially when the intake exceeds 750 mg day-1 26; However, this can be compensated through vitamin D and calcium ingestion at the recommended dose (800 mg day-1) 1,83 or through the intake of a glass of milk per day.26
Caffeine and alterations to the genetic code
Finally, although caffeine has a demonstrated in vitro mutagenic capacity 1,13 the evidence suggests that the alkaloid lacks mutagenic effects in humans. Caffeine lacks carcinogenic potential because it doesn't build complexes with DNA (adducts) since it doesn't covalently engage with it, is not metabolically activated and lacks the typical structural characteristics of purine-type carcinogens. In addition, in 1983, the Scientific Committee on Food concluded that based on evidence, caffeine doesn't exert mutagenic, carcinogenic or teratogenic effects in humans at current levels of consump-tion.55
Despite this, there are reports that caffeine can exacerbate the teratogenic capacity of tobacco and alcohol.1
Toxicity
Reports of fatalities caused by caffeine overdose are uncommon, and the registered cases aren't associated with the consumption of food or beverages rich in it but because of the ingestion of medications that contain it (Table 3).2,11,28,59 However, there are currently many recorded cases of acute toxicity due to the intake of energy drinks which, although they do not describe their caffeine content, may contain between 70 to 180 mg of the alkaloid per can of 8 to 16 ounces or 500 mg in 24-ounce bottles.59

Reproduced with permission of Graham 13 & McLellan et al. 60
Table 3. Over-the-Counter Medications that Contain Caffeine.
That is why the current US legislation limits caffeine content to 150 to 200 mg L-1 l-1 in all beverages unrelated to coffee or tea.12,22 In addition, to protect the consumer, the law mandates that all beverages unrelated to coffee or tea that exceed this limit should add the following note: Warning: Contains high amounts of caffeine.22
On the other side, caffeine's mean lethal dose (LD50) varies according to the individual susceptibility; however it is estimated to be ~ 200 mg kg-1 of live weight, which is tantamount to 3.5 g for an adult human being 60; The LD50 is a standard measure of the level of acute toxicity that is expressed in mg of substance per kg of live weight. The LD50 also represents the individual dose required to kill 50% of a population of test animals.
Generally speaking, the accepted LD50 oral is 10 g of caffeine,4,12,13 in other words, 170 mg kg-1 live weight 12,13, which is equivalent to drinking between 75 to 100 cups of coffee with the concomitant elevation of caffeine in blood plasma between 0.5 to 1 mM; However, there are recorded cases of deaths due to ingesting 6.5 g 102 or even 2 g of caffeine.2
Interestingly, taking advantage of its toxicity, there are records of the combined use of methylxanthines (i.e., caffeine and theobromine) in lethal doses for the demographic control of wild canine populations (e.g., coyotes).97
Toxicity manifests itself in sensitive adults in various ways such as nervousness, irritability, cardiovascular disorders (ie, cardiac arrhythmia [tachycardia] or cardiac arrest),9,11,19 insomnia, accelerated breathing, gastrointestinal disorders (e.g., nausea, vomiting, and diarrhea),11,19,102,103 diuresis,63,70,100,104 gastric acid secretion,63 seizures,13 elevated blood levels of free fatty acids and glucose,100 hypertension 65,69,105,106 and hypotension,11 resistance to the peripheral circulation,1 fever, hallucinations 11 and in the case of sensitive adults the consumption of 100 mg of caffeine (~ 1.4 mg kg-1 body weight) may reduce the duration of the sleep period,1,11,55,93 but the latter one can be mitigated by administering melatonin which is a natural hormone capable of regulating the sleep cycle.11
Although caffeine above mentioned disorders in human physiology depends on the speed with which it is absorbed, distributed, metabolized and excreted (through the urine), there is also evidence that certain disorders such as social anxiety disorder and panic disorder can exacerbate those conditions.82
Even though regular coffee consumption leads to developing some tolerance to several of these disorders (e.g., anxiety and increased heart rate) 2,11,28,81,86, the demand for decaffeinated coffees has gained terrain in the international market.
On the other hand, caffeine withdrawal can cause, in some individuals, a series of disorders that manifest approximately 20 to 40 h after the last consumption 11,28,82 among which we can mention: headaches, trouble concentrating, irritability, insomnia, weakness, anxiety, increased muscle tension, tremor, dizziness, nausea, and vomiting,26,28,59,100 fatigue and depression.11,26,28
Since disorders caused by caffeine withdrawal are relieved by consuming it again, some researchers propose that the beneficial physiological effects typically associated with caffeine (eg, ergogenic effect, improved attention, concentration, reflexes, and processing speed, improvement of long-term memory, etc.) are really due to the normalization of cognitive functions in individuals who have developed a dependence 59,60 who in turn develop a tolerance to caffeine reason why with time its dose tends to be gradually increased.67
However, regardless of regular consumption, there is evidence that the consumer's ability to develop caffeine tolerance is only partial or incomplete 82 in part due to their genomic constitution. For example, individuals with the allelic form CYP1A2*1F or CYP1A2 AA, both cytochrome P450, display a rapid caffeine metabolism 53,87,107; Meanwhile, individuals with other allelic variations such as CYP1A2 CC or CYP1A2*1K alleles display a slower alkaloid metabolism.85,87,107
Curiously, although caffeine was incorporated into the Manual of Diagnostics and Statistics of Mental Disorders (5th edition) of the American Psychiatric Association (APA), it has not yet been recognized as an addiction despite the apparent disorders associated with withdrawal in those individuals who have developed a dependence.11,28 This may be because the APA doesn't consider it a dangerous addiction for those who develop it 26, in addition to the fact that it has been referred to as a safe substance by the United States Department of Health and Human Services.4
Caffeine industrial applications
Annually, about 120 000 tm of caffeine are consumed 12, usually as an additive in cosmetics, cola drinks, and energy drinks 12,27,55 and in the particular case of cola soft drinks, caffeine is added to improve their flavor. In addition, caffeine is also used in the formulation of certain medications to relieve pain 28,65,82 in combination with anti-inflammatory analgesic formulas or with ergotamine for the treatment of migraine.12,103
There are also reports of its use together with ephedrine or synephrine in weight reduction treatments.55,103 Caffeine is also used as an additive in creams for the treatment of cellulitis due to its vasoconstrictor effect 27 which also reduces blood flow to the brain, which is why it is also used in the formulation of medications for the treatment of migraines or headaches.
CONCLUSION
When talking about caffeine, it is impossible not to talk about tea or coffee since both are plant sources that contain it in significant quantities and are the most consumed beverages worldwide. On the other hand, the fact that coffee appears as the second export item after oil and its derivatives renders the artificial synthesis of caffeine an eco-nomically irrelevant task.
Curiously, coffee occupies the second place in world consumption after tea 50,91,108 since the latter is consumed in many countries. According to Grigg 93 for every three cups of tea that are drunk in the world, one of coffee is consumed"; However, the cup of tea is cheaper than that of coffee because 1 kg of tea produces 4 to 5 times more cups than 1 kg of coffee.93
In addition, coffee's popularity is not based on its nutritional value or potential health benefits but on its pleasant taste and the stimulating properties of caffeine. This seems to be supported by the latest statistics published by the International Coffee Organization, according to which approximately 1.4 billion cups of coffee are consumed daily 50; Furthermore, the global consumption rate has doubled in the last 50 years because of the opening of new markets.11
Finally, the ever-increasing production is the primary reason for the current low prices of this commodity in the international market, independently of the species involved (C. arabica vs. C. canephora); As a consequence, large quantities of coffee and caffeine are available to the final consumers at very affordable prices. Thus, it can be concluded that coffee and, consequently, caffeine have managed to maintain their status to such an extent that no one considers a significant reduction in worldwide consumption despite religious restrictions such as the fact that since roasted coffee beans are similar in appearance to coal, its consumption is rohibited by the Koran.11
Funding: This research received no external funding.
Acknowledgments
The authors thank the Office for the Scientific, Humanistic and Technological Research (DICIHT) of the National Autonomous University of Honduras (UNAH) for their moral and technical assistantship during the preparation of this article.
Conflicts of Interest: The authors declare no conflict of interest.
REFERENCES
1.Greenberg J.A.; Boozer C.N.; Geliebter A. Coffee, diabetes, and weight control. Am J Clin Nutr. 2006;84(4):682-93. doi: 10.1093/ajcn/84.4.682.
2. Ludwig I.A.; Clifford M.N.; Lean ME; Ashihara H.; Crozier A. Coffee: biochemistry and potential impact on health. Food Funct. 2014;5(8):1695-717. doi: 10.1039/c4fo00042k.
3. Cano-Marquina A.; Tarin J.J.; Cano A. The impact of coffee on health. Maturitas. 2013;75(1):7-21. doi: 10.1016/j.maturitas.2013.02.002.
4. Pietsch A. Decaffeination - Process and quality. In: The craft and science of coffee. Folmer B, editor. Academic Press: 2017. p. 225-43. doi: 10.1016/b978-0-12-803520-7.00010-4.
5. Stefanello N.; Spanevello R.M.; Passamonti S.; Porciuncula L.; Bonan C.D.; Olabiyi A.A., et al. Coffee, caffeine, chlorogenic acid, and the purinergic system. Food Chem Toxicol. 2019; 123:298-313. doi: 10.1016/j.fct.2018.10.005.
6. Flament I.; Bessière-Thomas Y. Coffee flavor chemistry. 1 ed. John Wiley & Sons: West Sussex, ΡΟ19 1UD, England; 2001 November. doi: NO_DOI.
7. Miller M.G.; Shukitt-Hale B. Coffee and Alzheimer's disease: animal and cellular evidence. In: Coffee. Chu Y-F, editor. Wiley-Blackwell: Oxford, UK; 2012. p. 77-96. doi: 10.1002/9781119949893.ch4.
8. Ashihara H.; Monteiro A.M.; Gillies F.M.; Crozier A. Biosynthesis of caffeine in leaves of coffee. Plant Physiol. 1996;111(3):747-53. doi: 10.1104/pp.111.3.747.
9. Heckman M.A.; Weil J.; Gonzalez de Mejia E. Caffeine (1,3,7-trimethylxanthine) in foods: a comprehensive review on consumption, functionality, safety, and regulatory matters. J Food Sci. 2010;75(3):R77–R87. doi: 10.1111/j.1750-3841.2010.01561.x.
10. Herrera J.C.; Lambot C. The Coffee tree - Genetic diversity and origin. In: The Craft and Science of Coffee. Folmer B, editor. Academic Press: 2017. p. 1-16. doi: 10.1016/b978-0-12-803520-7.00001-3.
11. Folmer B.; Farah A.; Jones L.; Fogliano V. Human Wellbeing - Sociability, performance, and health. In: The craft and science of coffee. Folmer B, editor. Academic Press: 2017. p. 493-520. doi: 10.1016/b978-0-12-803520-7.00020-7.
12. Sereshti H.; Samadi S. A rapid and simple determination of caffeine in teas, coffees and eight beverages. Food Chem. 2014;158:8-13. doi: 10.1016/j.foodchem.2014.02.095.
13. Graham D.M. Caffeine - its identity, dietary sources, intake and biological effects. Nutr Rev. 1978;36(4):97-102. doi: 10.1111/j.1753-4887.1978.tb03717.x.
14. Franca A.S.; Mendonça J.C.F.; Oliveira S.D. Composition of green and roasted coffees of different cup qualities. LWT - Food Science and Technology. 2005;38(7):709-15. doi: 10.1016/j.lwt.2004.08.014.
15. Kreuml M.T.; Majchrzak D.; Ploederl B.; Koenig J. Changes in sensory quality characteristics of coffee during storage. Food Sci Nutr. 2013;1(4):267-72. doi: 10.1002/fsn3.35.
16. Joet T.; Laffargue A.; Salmona J.; Doulbeau S.; Descroix F.; Bertrand B., et al. Metabolic pathways in tropical dicotyledonous albuminous seeds: Coffea arabica as a case study. New Phytol. 2009;182(1):146-62. doi: 10.1111/j.1469-8137.2008.02742.x.
17. Pizzariello A.; vorc J.; Stred?ansky M.; Miertu S. A biosensing method for detection of caffeine in coffee. J Sci Food Agric. 1999;79(8):1136-40. doi: 10.1002/(sici)1097-0010(199906)79:8<1136::Aid-jsfa337>3.0.Co;2-4.
18. Farah A. Coffee constituents. In: Coffee. 1 ed. Chu Y-F, editor. Wiley-Blackwell: Oxford, UK; 2012. p. 21-58. doi: 10.1002/9781119949893.ch2.
19. Esquivel P.; Jiménez V.M. Functional properties of coffee and coffee by-products. Food Res Int. 2012;46(2):488-95. doi: 10.1016/j.foodres.2011.05.028.
20. Schenker S.; Rothgeb T. The Roast - Creating the beans' signature. In: The craft and science of coffee. Folmer B, editor. Academic Press: 2017. p. 245-71. doi: 10.1016/b978-0-12-803520-7.00011-6.
21. Poisson L.; Blank I.; Dunkel A.; Hofmann T. The chemistry of roasting - Decoding flavor formation. In: The craft and science of coffee. Folmer B, editor. Academic Press: 2017. p. 273-309. doi: 10.1016/b978-0-12-803520-7.00012-8.
22. Oestreich-Janzen S. Chemistry of coffee. In: Comprehensive natural products II. Elsevier: Oxford; 2010. p. 1085-117. doi: 10.1016/b978-008045382-8.00708-5.
23. Mussatto S.I.; Machado E.M.S.; Martins S.; Teixeira J.A. Production, composition, and application of coffee and its industrial residues. Food Bioproc Tech. 2011;4(5):661-72. doi: 10.1007/s11947-011-0565-z.
24. Wei F.; Tanokura M. Organic compounds in green coffee beans. In: Coffee in health and disease prevention. Academic Press: San Diego; 2015. p. 149-62. doi: 10.1016/b978-0-12-409517-5.00017-6.
25. Reineccius G. Natural flavoring materials. In: Source book of flavors. Reineccius G, editor. Springer US: Boston, MA; 1995. p. 176-364. doi: 10.1007/978-1-4615-7889-5_7.
26. O'Keefe J.H.; Bhatti S.K.; Patil H.R.; DiNicolantonio J.J.; Lucan S.C.; Lavie CJ Effects of habitual coffee consumption on cardiometabolic disease, cardiovascular health, and all-cause mortality. J Am Coll Cardiol. 2013;62(12):1043-51. doi: 10.1016/j.jacc.2013.06.035.
27. Mazzafera P. Which is the by-product: caffeine or decaf coffee? Food Energy Secur. 2012;1(1):70-75. doi: 10.1002/fes3.4.
28. Turnbull D.; Rodricks JV; Mariano G.F.; Chowdhury F. Caffeine and cardiovascular health. Regul Toxicol Pharmacol. 2017;89:165-85. doi: 10.1016/j.yrtph.2017.07.025.
29.Von Linné C. Biological significance of alkaloids. In: Alkaloids - Secrets of life. 143. 1 ed. Elsevier: Amsterdam; 2007. p. 141-80. doi: 10.1016/b978-044452736-3/50005-2.
30. Perrois C.; Strickler S.R.; Mathieu G.; Lepelley M.; Bedon L.; Michaux S., et al. Differential regulation of caffeine metabolism in Coffea arabica (Arabica) and Coffea canephora (Robusta). Planta. 2015;241(1):179-91. doi: 10.1007/s00425-014-2170-7.
31.Cheng B.; Furtado A.; Smyth H.E.; Henry R.J. Influence of genotype and environment on coffee quality. Trends Food Sci Technol. 2016; 57:20-30. doi: 10.1016/j.tifs.2016.09.003.
32. Zheng X.-Q.; Nagai C.; Ashihara H. Pyridine nucleotide cycle and trigonelline (N-methylnicotinic acid) synthesis in developing leaves and fruits of Coffea arabica. Physiol Plant. 2004;122(4):404-11. doi: 10.1111/j.1399-3054.2004.00422. x.
33.Noirot M.; Poncet V.; Barre P.; Hamon P.; Hamon S.; De Kochko A. Genome size variations in diploid African Coffea species. Ann Bot. 2003;92(5):709-14. doi: 10.1093/aob/mcg183.
34. McCarthy A.A.; McCarthy J.G. The structure of two N-methyltransferases from the caffeine biosynthetic pathway. Plant Physiol. 2007;144(2):879-89. doi: 10.1104/pp.106.094854.
35. Mayer J.L.S.; Carmello-Guerreiro S.M.; Mazzafera P. A functional role for the colleters of coffee flowers. AoB Plants. 2013;5(0): plt029-plt29. doi: 10.1093/aobpla/plt029.
36. Benatti L.B.; Silvarolla M.B.; Mazzafera P. Characterisation of AC1: a naturally decaffeinated coffee. Bragantia. 2012;71(2):143-54. doi: 10.1590/s0006-87052012000200001.
37. Charrier A.; Berthaud J. Botanical classification of coffee. In: Coffee. Clifford MN, Willson KC, editors. Springer US: 1985. p. 13-47. doi: 10.1007/978-1-4615-6657-1_2.
38. Lashermes P.; Combes M.C.; Robert J.; Trouslot P.; D'Hont A.; Anthony F., et al. Molecular characterisation and origin of the Coffea arabica L. genome. Mol Gen Genet. 1999;261(2):259-66. doi: 10.1007/s004380050965.
39. Pinto-Maglio C.A.F. Cytogenetics of coffee. Braz J Plant Physiol. 2006;18(1):37-44. doi: 10.1590/S1677-04202006000100004
40. Cramer P.J.S. A review of literature of coffee research in Indonesia. Inter-American Institute of Agricultural Sciences: Turrialba, Costa Rica; 1957 December. 262 p. doi: NO_DOI.
41. Aerts R.; Berecha G.; Gijbels P.; Hundera K.; Glabeke S.; Vandepitte K., et al. Genetic variation and risks of introgression in the wild Coffea arabica gene pool in south-western Ethiopian montane rainforests. Evol Appl. 2013;6(2):243-52. doi: 10.1111/j.1752-4571.2012.00285. x.
42.Tran H.T.; Lee L.S.; Furtado A.; Smyth H.; Henry R.J. Advances in genomics for the improvement of quality in coffee. J Sci Food Agric. 2016;96(10):3300-12. doi: 10.1002/jsfa.7692.
43. Hendre P.S.; Aggarwal R.K. DNA markers: development and application for genetic improvement of coffee. In: Genomics-assisted crop improvement. Varshney RK, Tuberosa R, editors. Springer Netherlands: 2007. p. 399-434. doi: 10.1007/978-1-4020-6297-1_15.
44. Pierozzi N.I. Orcein, C- and NOR-banding on mitotic chromosomes of some wild diploid coffee species (Coffea L.). Caryologia. 2013;66(1):76-83. doi: 10.1080/00087114.2013.787206.
45.Baumann T.W. Some thoughts on the physiology of caffeine in coffee: and a glimpse of metabolite profiling. Braz J Plant Physiol. 2006;18(1):243-51. doi: 10.1590/s1677-04202006000100017.
46. Silva R.M.G.; Brigatti J.G.F.; Santos V.H.M.; Mecina G.F.; Silva L.P. Allelopathic effect of the peel of coffee fruit. Sci Hortic (Amsterdam). 2013; 158:39-44. doi: 10.1016/j.scienta.2013.04.028.
47. Ransom F. The effects of caffeine upon the germination and growth of seeds. Biochem J. 1912;6(2):151-55. doi: NO_DOI.
48. Charrier A.; Eskes A.B. Les caféiers. In: L'Amélioration des plantes tropicales. Charrier A, Jacquot M, Hamon S, Dominique N, editors. CIRAD et ORSTOM: Paris; 1997. p. 171–96. doi: NO_DOI.
49. Pierozzi N.I.; Borghi T.C.; Silvarolla M.B. A karyological study in some species of Coffea L. and in the closest relative Psilanthus travancorensis (Wight & Arn.) J.-F. Leroy. Not Bot Horti Agrobot Cluj Napoca. 2012;40(2):39-45. doi: 10.15835/nbha4028195.
50. Caprioli G.; Cortese M.; Sagratini G.; Vittori S. The influence of different types of preparation (espresso and brew) on coffee aroma and main bioactive constituents. Int J Food Sci Nutr. 2015;66(5):505-13. doi: 10.3109/09637486.2015.1064871.
51. Komes D.; Bušić A. Antioxidants in coffee. In: Processing and impact on antioxidants in beverages. Preedy V, editor. Academic Press: San Diego; 2014. p. 25-32. doi: 10.1016/b978-0-12-404738-9.00003-9.
52. McCusker R.R.; Goldberger B.A.; Cone E.J. Caffeine content of specialty coffees. J Anal Toxicol. 2003;27(7):520-22. doi: 10.1093/jat/27.7.520.
53. Chan R.L. Epidemiological evidence for maternal prenatal coffee and caffeine consumption and miscarriage risk. In: Coffee. Chu Y-F, editor. Wiley-Blackwell: Oxford, UK; 2012. p. 243-58. doi: 10.1002/9781119949893.ch14.
54.Tfouni S.A.V.; Carreiro L.B.; Teles CRA; Furlani R.P.Z.; Cipolli KMVAB; Camargo M.C.R. Caffeine and chlorogenic acids intake from coffee brew: influence of roasting degree and brewing procedure. Int J Food Sci Technol. 2014;49(3):747-52. doi: 10.1111/ijfs.12361.
55. EFSA Panel on Dietetic Products N.a.A. Scientific opinion on the safety of caffeine. EFSA Journal. 2015;13(5):1-120. doi: 10.2903/j.efsa.2015.4102.
56. Guallar E.; Blasco-Colmenares E.; Arking DE; Zhao D. Moderate coffee intake can be part of a healthy diet. Ann Intern Med. 2017;167(4):283-84. doi: 10.7326/M17-1503.
57. Folwarczna J.; Janas A.; Pytlik M.; Cegiela U.; Sliwinski L.; Krivosikova Z., et al. Effects of trigonelline, an alkaloid present in coffee, on diabetes-induced disorders in the rat skeletal system. Nutrients. 2016;8(3):133. doi: 10.3390/nu8030133.
58. Folwarczna J.; Zych M.; Nowinska B.; Pytlik M.; Janas A. Unfavorable effect of trigonelline, an alkaloid present in coffee and fenugreek, on bone mechanical properties in estrogen-deficient rats. Mol Nutr Food Res. 2014;58(7):1457-64. doi: 10.1002/mnfr.201300936.
59. Childs E.; de Wit H. Potential mental risks. In: Coffee. Chu Y-F, editor. Wiley-Blackwell: Oxford, UK; 2012. p. 293-306. doi: 10.1002/9781119949893.ch17.
60. McLellan T.M.; Caldwell J.A.; Lieberman H.R. A review of caffeine's effects on cognitive, physical and occupational performance. Neurosci Biobehav Rev. 2016; 71:294-312. doi: 10.1016/j.neubiorev.2016.09.001.
61. Homan D.J.; Mobarhan S. Coffee: good, bad, ¿or just fun? a critical review of coffee's effects on liver enzymes. Nutr Rev. 2006;64(1):43-46. doi: 10.1111/j.1753-4887. 2006.tb00172. x.
62. Cadden I.S.; Partovi N.; Yoshida E.M. Possible beneficial effects of coffee on liver disease and function. Aliment Pharmacol Ther. 2007;26(1):1-8. doi: 10.1111/j.1365-2036.2007.03319. x.
63. Trugo L.C.; Macrae R.; Dick J. Determination of purine alkaloids and trigonelline in instant coffee and other beverages using high performance liquid chromatography. J Sci Food Agric. 1983;34(3):300-06. doi: 10.1002/jsfa.2740340314.
64. Dessalegn Y.; Labuscagne M.T.; Osthoff G.; Herselman L. Variation of green bean caffeine, chlorogenic acid, sucrose and trigolline contents among Ethiopian Arabica coffee accessories. SINET: Ethiop J Sci. 2008;30(1):77-82. doi: 10.4314/sinet. v30i1.18286.
65. Farah A.; de Paulis T.; Moreira D.P.; Trugo L.C.; Martin P.R. Chlorogenic acids and lactones in regular and water-decaffeinated arabica coffees. J Agric Food Chem. 2006;54(2):374-81. doi: 10.1021/jf0518305.
66. Narita Y.; Inouye K. Chlorogenic acids from coffee. In: Coffee in health and disease prevention. Academic Press: San Diego; 2015. p. 189-99. doi: 10.1016/b978-0-12-409517-5.00021-8.
67. Rubach M.J.; Somoza V. Impact of coffee on gastric acid secretion. In: Coffee. Chu Y-F, editor. Wiley-Blackwell: Oxford, UK; 2012. p. 275-91. doi: 10.1002/9781119949893.ch16.
68. Siebert M.; Berger R.G.; Nieter A. Enzymatic mitigation of 5-O-chlorogenic acid for an improved digestibility of coffee. Food Chem. 2018; 258:124-28. doi: 10.1016/j.foodchem.2018.03.061.
69. Smits P.; Thien T.; Van 't Laar A. The cardiovascular effects of regular and decaffeinated coffee. Br J Clin Pharmacol. 1985;19(6):852-54. doi: 10.1111/j.1365-2125. 1985.tb02729. x.
70. Turnbull A.J. Coffee. Nutr Bull. 1981;6(3):153-65. doi: 10.1111/j.1467-3010. 1981.tb00494. x.
71. Mishra M.K.; Slater A. Recent advances in the genetic transformation of coffee. Biotechnol Res Int. 2012; 2012:580857. doi: 10.1155/2012/580857.
72.Farah A.; Donangelo C.M. Phenolic compounds in coffee. Braz J Plant Physiol. 2006;18(1):23-36. doi: 10.1590/s1677-04202006000100003.
73. de Azevedo A.B.A.; Kieckbush T.G.; Tashima A.K.; Mohamed R.S.; Mazzafera P.; Melo S.A.B.V.d. Extraction of green coffee oil using supercritical carbon dioxide. J Supercrit Fluids. 2008;44(2):186-92. doi: 10.1016/j.supflu.2007.11.004.
74. Couto R.M.; Fernandes J.; da Silva MDRG; Simões P.C. Supercritical fluid extraction of lipids from spent coffee grounds. J Supercrit Fluids. 2009;51(2):159-66. doi: 10.1016/j.supflu.2009.09.009.
75. Ahangari B.; Sargolzaei J. Extraction of lipids from spent coffee grounds using organic solvents and supercritical carbon dioxide. J Food Process Preserv. 2013;37(5):1014-21. doi: 10.1111/j.1745-4549.2012.00757. x.
76. van Dam J.E.G.; Harmsen P. Coffee residues utilization. Report. Wageningen UR Food & Biobased Research, VLAG, Technology FBBNF; 2010. Disponible en: NO_DOI.
77. Chen Y.; Brown P.H.; Hu K.; Black R.M.; Prior RL; Ou B., et al. Supercritical CO2 decaffeination of unroasted coffee beans produces melanoidins with distinct NF‐κB inhibitory activity. J Food Sci. 2011;76(7):H182–H86. doi: 10.1111/j.1750-3841.2011.02304. x.
78. Ky CL; Louarn J.; Dussert S.; Guyot B.; Hamon S.; Noirot M. Caffeine, trigonelline, chlorogenic acids and sucrose diversity in wild Coffea arabica L. and C. canephora P. accessions. Food Chem. 2001;75(2):223-30. doi: 10.1016/s0308-8146(01)00204-7.
79. Muriel P.; Arauz J. Coffee and liver health. In: Coffee. Chu Y-F, editor. Wiley-Blackwell: Oxford, UK; 2012. p. 123-39. doi: 10.1002/9781119949893.ch7.
80. Chu Y.-F.; Chen Y. Additional positive impacts on health. In: Coffee. Chu Y-F, editor. Wiley-Blackwell: Oxford, UK; 2012. p. 233-41. doi: 10.1002/9781119949893.ch13.
81. Chrysant S.G. Coffee consumption and cardiovascular health. Am J Cardiol. 2015;116(5):818-21. doi: 10.1016/j.amjcard.2015.05.057.
82. Grant S.S.; Magruder K.P.; Friedman B.H. Controlling for caffeine in cardiovascular research: a critical review. Int J Psychophysiol. 2018; 133:193-201. doi: 10.1016/j.ijpsycho.2018.07.001.
83. Bae J.H.; Park J.H.; Im S.S.; Song DK Coffee and health. Integr Med Res. 2014;3(4):189-91. doi: 10.1016/j.imr.2014.08.002.
84. Lean ME; Crozier A. Coffee, caffeine and health: ¿what’s in your cup? Maturitas. 2012;72(3):171-72. doi: 10.1016/j.maturitas.2012.04.005.
85. O'Keefe J.H.; DiNicolantonio J.J.; Lavie CJ Coffee for cardioprotection and longevity. Prog Cardiovasc Dis. 2018;61(1):38-42. doi: 10.1016/j.pcad.2018.02.002.
86. Musgrove C. Coffee - the truth about the bean. Nutr Bull. 2006;31(4):282-85. doi: 10.1111/j.1467-3010.2006.00587. x.
87. Cornelis M.C. Gene-coffee interactions and health. Curr Nutr Rep. 2014;3(3):178-95. doi: 10.1007/s13668-014-0087-1.
88. Chen S.; Teoh NC; Chitturi S.; Farrell G.C. Coffee and non-alcoholic fatty liver disease: brewing evidence for hepatoprotection? J Gastroenterol Hepatol. 2014;29(3):435-41. doi: 10.1111/jgh.12422.
89. Jeszka-Skowron M.; Stanisz E.; De Peña M.P. Relationship between antioxidant capacity, chlorogenic acids and elemental composition of green coffee. Lwt. 2016; 73:243-50. doi: 10.1016/j.lwt.2016.06.018.
90. Buscemi S.; Marventano S.; Antoci M.; Cagnetti A.; Castorina G.; Galvano F., et al. Coffee and metabolic impairment: an updated review of epidemiological studies. NFS Journal. 2016;3:1-7. doi: 10.1016/j.nfs.2016.02.001.
91. Watawana M.I.; Jayawardena N.; Waisundara V.Y. Enhancement of the functional properties of coffee through fermentation by "Tea Fungus" (Kombucha). J Food Process Preserv. 2015;39(6):2596-603. doi: 10.1111/jfpp.12509.
92. Kennedy O.J.; Roderick P.; Buchanan R.; Fallowfield JA; Hayes P.C.; Parkes J. Systematic review with meta-analysis: coffee consumption and the risk of cirrhosis. Aliment Pharmacol Ther. 2016;43(5):562-74. doi: 10.1111/apt.13523.
93. Grigg D. The worlds of tea and coffee: patterns of consumption. GeoJournal. 2002;57(4):283-94. doi: 10.1023/B:GEJO.0000007249.91153.c3.
94. Ribeiro V.S.; Leitão A.E.; Ramalho J.C.; Lidon F.C. Chemical characterization and antioxidant properties of a new coffee blend with cocoa, coffee silverskin and green coffee minimally processed. Food Res Int. 2014;61:39-47. doi: 10.1016/j.foodres.2014.05.003.
95. Lopez-Garcia E.; Guallar-Castillon P.; Leon-Munoz L.; Graciani A.; Rodriguez-Artalejo F. Coffee consumption and health-related quality of life. Clin Nutr. 2014;33(1):143-49. doi: 10.1016/j.clnu.2013.04.004.
96. Lim J.-W.; Tan E.-K. Coffee and Parkinson's disease. In: Coffee. Chu Y-F, editor. Wiley-Blackwell: Oxford, UK; 2012. p. 111-22. doi: 10.1002/9781119949893.ch6.
97. Delfiol D.J.; Oliveira-Filho J.P.; Casalecchi F.L.; Kievitsbosch T.; Hussni C.A.; Riet-Correa F., et al. Equine poisoning by coffee husk (Coffea arabica L.). BMC Vet Res. 2012;8(1):4. doi: 10.1186/1746-6148-8-4.
98. Reis C.E.G.; Dórea J.G.; da Costa T.H.M. Effects of coffee consumption on glucose metabolism: a systematic review of clinical trials. J Tradit Complement Med. 2019;9(3):184-91. doi: 10.1016/j.jtcme.2018.01.001.
99. Morris J. We consumers - Tastes, rituals, and waves. In: The craft and science of coffee. Folmer B, editor. Academic Press: 2017. p. 457-91. doi: 10.1016/b978-0-12-803520-7.00019-0.
100. McCusker R.R.; Fuehrlein B.; Goldberger B.A.; Gold M.S.; Cone E.J. Caffeine content of decaffeinated coffee. J Anal Toxicol. 2006;30(8):611-13. doi: 10.1093/jat/30.8.611.
101. Pehl C.; Pfeiffer A.; Wendl B.; Kaess H. The effect of decaffeination of coffee on gastro-oesophageal reflux in patients with reflux disease. Aliment Pharmacol Ther. 1997;11(3):483-86. doi: 10.1046/j.1365-2036.1997.00161.x.
102. Nawrot P.; Jordan S.; Eastwood J.; Rotstein J.; Hugenholtz A.; Feeley M. Effects of caffeine on human health. Food Addit Contam. 2003;20(1):1-30. doi: 10.1080/0265203021000007840.
103. Nurminen M.L.; Niittynen L.; Korpela R.; Vapaatalo H. Coffee, caffeine and blood pressure: a critical review. Eur J Clin Nutr. 1999;53(11):831-39. doi: 10.1038/sj.ejcn.1600899.
104. Graham T.E.; Hibbert E.; Sathasivam P. Metabolic and exercise endurance effects of coffee and caffeine ingestion. J Appl Physiol (1985). 1998;85(3):883-89. doi: 10.1152/jappl.1998.85.3.883.
105. Roach R.E.; Siegerink B.; le Cessie S.; Rosendaal F.R.; Cannegieter S.C.; Lijfering W.M. Coffee consumption is associated with a reduced risk of venous thrombosis that is mediated through hemostatic factor levels. J Thromb Haemost. 2012;10(12):2519-25. doi: 10.1111/jth.12034.
106. Hammar N.; Andersson T.; Alfredsson L.; Reuterwall C.; Nilsson T.; Hallqvist J., et al. association of boiled and filtered coffee with incidence of first nonfatal myocardial infarction: the SHEEP and the VHEEP study. J Intern Med. 2003;253(6):653-59. doi: 10.1046/j.1365-2796.2003.01142.x.
107. Thorn C.F.; Aklillu E.; Klein T.E.; Altman R.B. PharmGKB summary: very important pharmacogene information for CYP1A2. Pharmacogenet Genomics. 2012;22(1):73-77. doi: 10.1097/FPC.0b013e32834c6efd.
108. Dasgupta A.; Klein K. Tea, coffee, and chocolate. In: Antioxidants in food, vitamins and supplements. Elsevier: San Diego; 2014. p. 237-57. doi: 10.1016/b978-0-12-405872-9.00013-6.
Received: 20 March 2022 / Accepted: 25 july 2022 / Published:15 Agoust 2022
Citation: Portillo O R, Arévalo A C. Caffeine. A critical review of contemporary scientific literature. Revis Bionatura 2022;7(3) 16. http://dx.doi.org/10.21931/RB/2022.07.03.16