2023.08.03.90
Files > Volume 8 > Vol 8 No 3 2023

Effects of various xenogenic mesenchymal stem cell secretome fractions on the regenerative capacity of the liver in vitro
1 V.N. Karazin Kharkiv National University, Kharkiv, Ukraine;
* Correspondence: [email protected]
Available from: http://dx.doi.org/10.21931/RB/2023.08.03.90
ABSTRACT
Liver diseases cause many deaths worldwide and wreak havoc on the economy. The main hepatoprotectors are plant substances and peptides. Regenerative medicine based on mesenchymal stem cells (MSCs) can offer a new set of biologically active substances for liver regeneration, which are part of their secretome. The work applied the methods of cell isolation and cultivation, ultrafiltration for fractionation of secretome components, and organotypic culture model. The study’s results indicate an increase in the regenerative capacity of the liver under the impact of the components of the MSC secretome; the fraction below 10 kDa shows the most significant activity.
Keywords: organotypic liver culture; xenogenic stem cells; fractions of secretome; absorption spectrum, ultrafiltration
INTRODUCTION
Annually, liver diseases claim the lives of 2 million people worldwide, making this group of diseases the 11th most common cause of death. About 2 billion people worldwide consume alcohol, resulting in 75 million cases of liver disease. The exact number of adults are obese or overweight, and more than 400 million have diabetes, which carries the risk of non-alcoholic fatty liver disease and hepatocellular carcinoma 1. The high prevalence and danger of liver pathology occasion a wide range of hepatoprotectors. The most common hepatoprotection are compositions based on herbal raw material, for example, rooibos tea (Aspalathus linearis), Elephantopus scaber Linn. and silymarin isolated from Saint-Mary-thistle (Silybum marianum) 2–4, as well as an amino acid mixture, peptides, e.g., natural, isolated from oyster, mealworm, or synthetic S-adenosyl-L-methionine 5–7. At the same time, the available means for preventing and treating liver diseases are not always effective enough, especially in the case of severe fibrotic changes, which stimulates the search for new, more active hepatoprotectors.
Renovative medical technologies using mesenchymal stem cells (MSCs) show good progress in the recovery of damaged liver tissues. MSCs are pluripotent cells derived from various sources, including bone marrow, adipose tissue, and cord blood. They can proliferate, differentiate and home. However, using living cells has several limitations, including, in particular, the risks of incomplete or incorrect cell differentiation, malignancy, pathological angiogenesis, and improper targeted delivery, which leads to small vessel thrombosis 8,9.
The described limitations stimulate interest in technologies based on stem cell exometabolites (secretome) rather than living cells. The secretome of MSCs is predominantly soluble proteins, including cytokines, chemokines, growth factors, and proteolytic enzymes, as well as extracellular vesicles that can express surface markers characteristic of MSCs and contain antifibrotic and antiapoptotic proteins or specific regulatory RNAs 10.
The biological effects of cells administrated into the body are typical for different organs and come down to reducing inflammation, modulating the immune system, reducing oxidative stress, affecting the synthesis and / or destruction of extracellular matrix proteins, as well as activating the division and differentiation of resident stem cells of damaged organs 9–11. They are caused by the paracrine action of MSCs and their exometabolites 12.
Most of the molecules that ensure liver regeneration belong to the high molecular weight fraction of the secretome (over 30 kDa). There needs to be more information about the low molecular weight components of the conditioned medium of MSCs. There is data on the effects of individual miRNAs 9; however, a comprehensive study of the combined action of low molecular weight regulatory RNAs and peptides has yet to be carried out. In the present study, the research on the biological activity of individual fractions of the MSC secretome, separated by their weight, was carried out. This approach is novel; it will allow us to determine the most active fraction for subsequent detailed investigation of the mechanisms of its action, and the study results can be applied in biotechnology to develop hepatoprotectors.
This study aimed to research the rat liver’s regenerative capacity in vitro using an organotypic culture model under the influence of the whole secretome of xenogenic mesenchymal stem cells and its individual fractions separated by mass by ultrafiltration.
MATERIALS AND METHODS
Obtaining a conditioned medium
Mesenchymal stem cells (MSCs) from the bone marrow of xenogeneic origin were obtained using the standard method 13. Biomaterial sampling from a healthy donor animal (dog) in the quantity of 10 ml was performed by puncture from the femur under epidural anesthesia with consecutive placing in a phosphate-buffered saline solution PanEcoTM. The obtained tissue samples under aseptic conditions of a laminar-flow box “Laminar systems” were washed with liquid nutrient medium DMEM high glucose PanEcoTM; the resulting bone marrow suspension was disaggregated and plated in a culture flask TPP in growth medium 90% DMEM, 10% fetal bovine blood serum BioloTTM, 50 μg/ml gentamicin SINTEZTM and amphotericin MICROGENTM. Incubation was carried out at 37 ºC and 5% CO2 in the atmosphere by dint CO2 incubator Binder. Twenty-four hours after cell isolation, the floating fraction was removed. The attached plastic-adhesive cells are MSCs. Compliance of the obtained cells with the criteria for belonging to MSCs (plastic-adhesiveness, ability to differentiate in at least three directions (osteo-, chondro- and adipogenic) and cellular immunophenotype) is ensured by the exact implementation of the method of their isolation.
The cells of the second passage (Fig. 1a) were cryopreserved according to the standard method 14. The cells were removed from the substrate using a dispersing solution of 0.05% of trypsin PanEco in Versen’s solution PanEcoTM, plated into a cryoprotective medium contented 90% of fetal serum and 10% of DMSO PanEcoTM, manufactured in the United States, distributed between cryovials SPL LifesciencesTM and cooled at a rate of about 1 ºC/min. The culture was restored after freezing (Fig. 1b) in DMEM, a high glucose nutrient medium supplemented with 10% fetal bovine blood serum and cultivated as before. After the second passage, the growth medium was replaced with a maintenance medium (with a content of serum reduced to 2 %). At 48 hours of culture growth, a conditioned medium containing the secretome of mesenchymal stem cells was collected. The whole fraction of the secretome was purified from cell detritus using MPW-56/10056 centrifuge and used in further research.
MSC secretome fractionation
MSC secretome fractionation was performed by ultrafiltration on a laboratory filtration unit (Fig. 1c) consisting of a Masterflex peristaltic pump and Pellicon (Biomax) ultrafiltration cassettes with a pore rating of 10 and 30 kDa (used alternately) interconnected by silicone tubes. Firstly, two fractions were obtained from the conditioned medium: a concentrate (below 10 kDa) and a permeate (over 10 kDa). Then, the permeate from the first stage was ultrafiltered on a cassette with pores of 30 kDa, which made it possible to obtain a fraction of 10–30 kDa (concentrate) and a fraction with a molecular weight of over 30 kDa (permeate). Thus, three fractions were obtained in addition to the whole conditioned medium containing the entire spectrum of exometabolites: below 10 kDa, 10–30 kDa and over 30 kDa. A supporting medium with 98% DMEM and 2% fetal bovine blood serum was used as a control.
Spectrophotometric study
The composition of the conditioned medium and its fractions was estimated by analyzing the absorption spectrum on a UV-2600 double-beam spectrophotometer Shimadzu, taken in the range from 200 to 400 nm; a blank sample was DMEM medium. Based on the spectra obtained by standard methods (D280/D260 & V. F. Kalb, R. W. Bernlohr methods for proteins and D280/D260 for nucleic acids) 15, the content of protein and nucleic acids in the samples was determined.
Model of organotypic liver culture
The test was performed according to method 16, modified by the author. The liver was removed from Wistar rats (males weighing at least 200 g, at least 3 months old), decapitated after thiopental anesthesia, and dissected. Several fragments about 1 x 1 cm in size were excised from different parts of the organ and placed in a solution of 0.9% sodium chloride Yuriya-PharmTM. The organ fragments were cooled to a temperature of +2...8 °C; after that, they were transferred to a laboratory box and, following the rules of asepsis, using a prepared metal round in cross-section sampler, explants were isolated and placed in sterile plastic Petri dishes BiosigmaTM on a nutrient medium consisting of 90% DMEM/F12 medium and 10% fetal bovine blood serum BiowestTM, as well as gentamicin ZdorovyeTM and amphotericin BioloTTM at 50 μg/ml. The culture was incubated for 48 hours at 37.0 ± 0.5 °C under hypoxia and elevated carbon dioxide concentration. After incubation, the experiment was considered by processing the culture images obtained by dint microscopy (microscope BiolamTM, magnification × 100). Image processing was carried out in the ToupViewTM 4.11 program (Fig. 1d) by determining the area of the central (dark) zone (explant zone) and the peripheral (light) zone (exclusion and growth zone). After that, the area index (AI) is calculated using the formula:

CZ is the central zone (explant) area, and GZ is the exclusion and growth zone in mm2 or pixels.
The adhesiveness index was also considered the percentage of explants attached to the substrate at the end of incubation.
The test substances were administrated into the growth medium where the explants were cultivated. It is also possible to study the change in the parameters of the expansion of liver cells under the influence of factors that acted on the animal’s body from which the explants were obtained.
The choice of concentrations was because a pilot study of the activity of the whole secretome in rats, which confirmed its effectiveness concerning regenerative processes, was centered on a dosage of 50 μl/100 g of body weight. Taking into account the bioavailability of substances administered intramuscularly (at least 80%) and the volume of circulating blood of rats (about 60 ml), the estimated effective dose was set as 60 μl/ml. To identify the expected dose dependence of the biological effect, smaller and larger doses were taken – 30 and 120 μl/ml.
Statistical data analysis
Statistical data analysis was carried out in stages using the Excel 2016 and STATISTICA 12 software packages according to standard methods 17,18. In the first stage, the distributions in the samples were tested for normality by determining the significance of differences in the coefficients of kurtosis and skewness from 0. After that, the Sheff multiple comparison method or its non-parametric analogs (based on Friedman ranking) were used. To determine the effect of the concentration of secretome on the intensity of the formation of the zone of eviction and growth, the methods of correlation analysis were applied (Pearson’s criterion or its non-parametric analog, Spearman’s criterion). All criteria were applied under standard conditions (power should be at least 80%, and the significance level was higher than 0.05).
Ethics statement
All manipulations with animals complied with international legislation requirements for the protection of vertebrate animals 19, and the Bioethics Commission approved the study. The study protocol and report were approved at meetings of the Bioethical Commission of the Department of Molecular Biology and Biotechnology of the Faculty of Biology of V.N. Karazin Kharkiv National University.
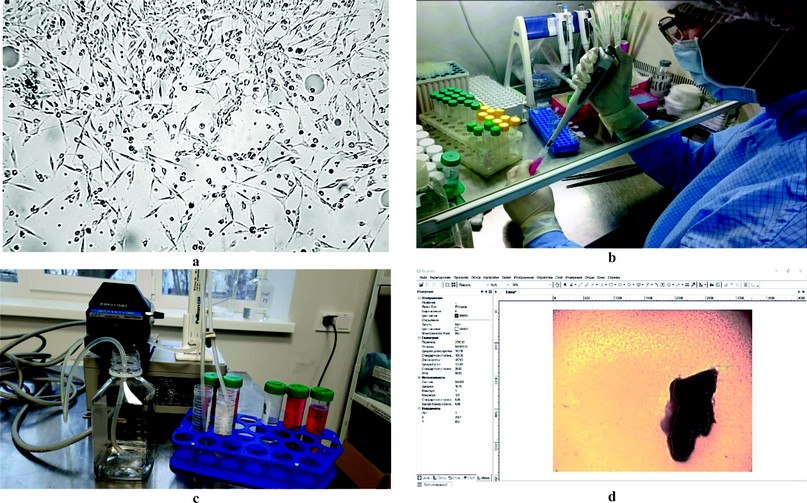
Figure 1. Research Methods: (a) Culture of dog mesenchymal stem cells under an inverted microscope (magnification × 100); (b) Restore of cell culture after cryopreservation; (c) Separation of the conditioned medium into fractions; (d) Taking into account the intensity of the formation of the zone of exclusion and growth.
RESULTS
Spectrophotometric assessment of the composition of the secretome and its fractions
To assess the composition of the obtained MSCs secretome fractions, the absorption spectrum of the samples in the range of 200-400 nm was analyzed (Fig. 2). When analyzing the spectrum, the concentrations of protein and nucleic acids were determined (Fig. 3), and a cluster analysis with using STATISTICA 12 was performed for 21 points of the spectrum, which were “peaks” or “dips” (Fig. 4).
The absorption spectrum of the control, whole conditioned medium containing MSC secretion, as well as its fractions, differ. The most significant differences characterize the fraction with a molecular weight over 30 kDa.
The composition of the nutrient medium (control) contains bovine serum albumin and amino acids, which have absorption spectra in 230 and 260 nm, similar to biologically active proteins and peptides produced by MSCs. The spectrogram in Figure 2 clearly shows that the whole conditioned medium in which MSCs were cultivated (red line) contains a more significant amount of peptides. These proteins absorb at 260 nm than the control (black line), because they have a higher optical density in this region. The first peak (region 230 nm) is higher and shifted to the right, indicating a different amino acid composition of the peptides from the control.
The absorption spectra of MSC secretome fractions are very different, both from the control and the whole conditioned medium containing exometabolites. Fractions less than 10 kDa (blue line) and 10-30 kDa (green line) contain a much smaller number of chromatophores compared to whole conditioned medium and control (Fig. 2), which is associated with the absence of high molecular weight proteins, in the low molecular weight fraction (blue line), peaks are observed in the region of 220-230 nm, corresponding to peptides with sulfur-containing amino acids (cystine, cysteine, methionine), and in the region of 280 nm, which is associated with peptides containing aromatic amino acids; peaks are also observed at 240 and 340 nm (of the amino acids that absorb in the wavelength range of 240-340 nm, tryptophan has the most intense light absorption, followed by tyrosine, phenylalanine and cystine); the presence of metal-containing domains (Zn, Cu, etc.) in the composition of these peptides are also possible, which provide a change in the intensity of adsorption in these parts of the spectrum. The 10-30 kDa fraction (green line) is smaller than peptides with sulfur-containing amino acids (220-230 nm) and more significant than those containing aromatic amino acids (240 nm). It should be noted that both considered fractions do not contain significant peaks in the region of 260 nm associated with nucleic acids. This is probably because regulatory miRNAs are small and act at low concentrations, so they cannot be captured by such a crude method.
The high-molecular part of the secretome (weighing over 30 kDa) does not contain low-molecular regulatory peptides, as indicated by dips in the region of 220-230 and 260 nm (Fig. 2, purple line). At the same time, the fraction contains a certain amount of high molecular weight proteins (zone 280 nm and beyond), and their relative concentration is higher than in other fractions. The chromatophores of this region, purified from low-molecular impurities, exhibit more intense absorption.
Analysis of the spectrograms showed a relatively low content of nucleic acids in the secretome fractions since it is a strong chromatophore in the region of 260 nm, and the fractions of the conditioned medium containing exometabolites did not show a peak in this region.
Comparison of two methods for determining protein concentration (D280/D260 and V. F. Kalb, R. W. Bernlohr methods) using the non-parametric Spearman test showed a high significant correlation between them (r=0.9, p≤0.05). However, the D280/D260 method is probably more sensitive, as pointed out in the difference in this indicator between the control sample, which does not contain exometabolites, and the whole conditioned medium, in which the components of the secretome of MSCs were accumulated (Fig. 3).
Determination of the content of nucleic acids showed its slight excess in the whole conditioned medium and a more significant increase in various fractions. Notably, the maximum concentration of nucleic acids was in variants with a molecular weight of 10–30 kDa and over 30 kDa (Fig. 3).
As seen in Figure 2, the absorption spectrum of the control, whole conditioned medium containing MSC secretome and its fractions differ. The most significant differences are characterized by a fraction with a molecular weight over 30 kDa. The resulting spectra can be used to confirm that the product belongs to a particular secretome fraction in production and screening studies.
Visible differences in spectra were confirmed by statistical analysis of 21 points of each spectrum, which were “peaks” or “dips .” For each sample, the optical density was determined at the indicated points. Based on these values, using the STATISTICA 12 program, a multidimensional scaling graph was constructed, which made it possible to distinguish three clusters according to the similarity of the absorption spectrum: cluster 1 – control and the whole fraction of the conditioned medium; cluster 2 – fractions weighing below 10 kDa and 10–30 kDa, and cluster 3 – a fraction weighing over 30 kDa (Fig. 4).
As can be seen, during ultrafiltration, some of the optically active components contained in the initial nutrient medium (control, sample without secretome) and remaining in the whole secretome are lost. The lowest molecular weight fraction (below 10 kDa) and the 10–30 kDa fraction have a set of substances distinguishing them from the high molecular weight fraction (over 30 kDa) and the initial medium.
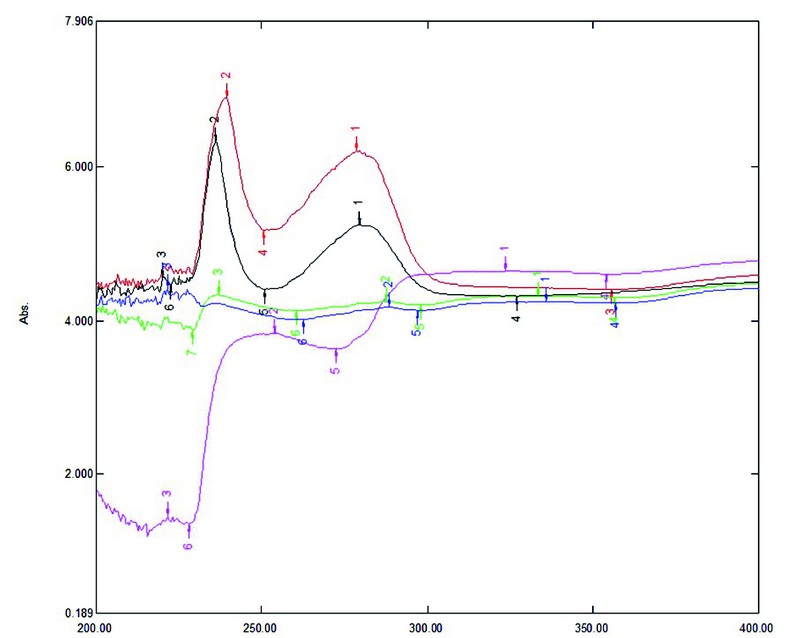
Figure 2. Absorption spectrum of growth medium containing different fractions of exometabolites of dog mesenchymal stem cells, obtained by ultrafiltration, taken in the range from 200 to 400 nm: black – control (without exometabolites); red – whole conditioned medium; blue – below 10 kDa; green – from 10 to 30 kDa; lilac – over 30 kDa.
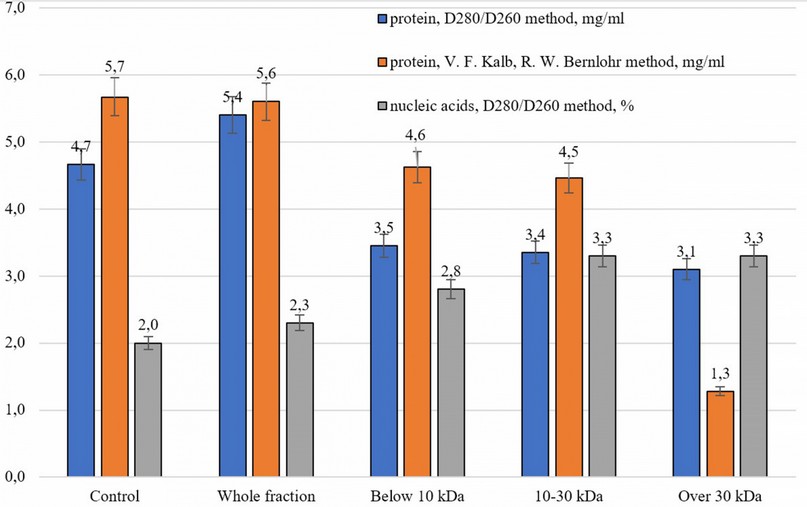
Figure 3. The concentration of protein and nucleic acids in the studied samples
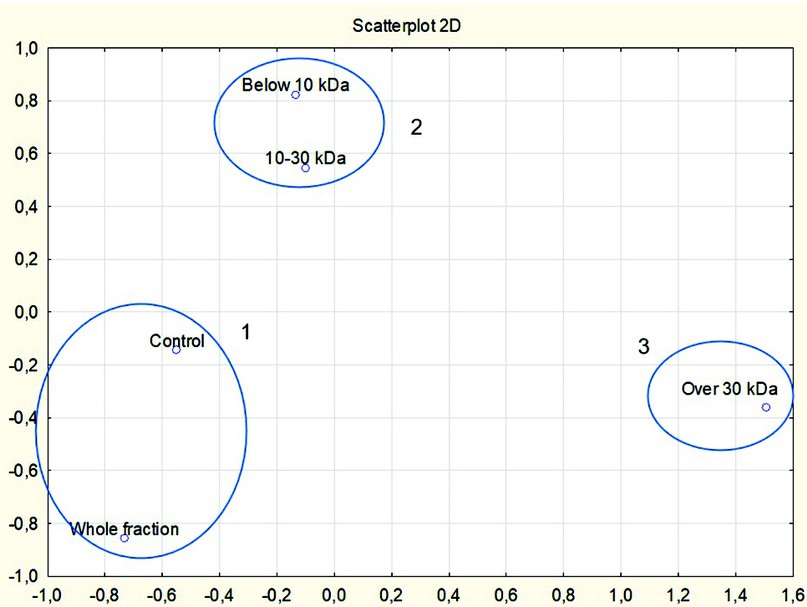
Figure 4. Clusters based on multidimensional scaling of the spectrum of samples carried out over 21 points in the range from 202 to 400 nm
The biological effect of the whole secretome
At the first stage of the biological activity research, a whole conditioned medium containing secretome (exometabolites) produced by MSCs was studied. Concentrations of 30, 60, and 120 μl/ml (in terms of protein, 105, 210, and 420 μg/ml, respectively) were studied; the control was a maintenance medium without secretome components. (Fig. 5).
As shown in Figure 5, all experimental variants formed more intensively the zone of exclusion and growth than the control. The excess of the area index by 2.3–3.4 times was statistically significant (p ≤ 0.01).
Correlation analysis (Fig. 5a) revealed a strong relationship between the concentration of the conditioned medium and the observed biological effect (r = 0.78; p ≤ 0.01). In the STATISTICA program, the following equation was obtained that describes the studied dependence:

where AI is the area index, %, and C is the concentration of the conditioned medium, µl/ml
With the help of a graph built in Excel based on the medians of the samples, a trend line with a polynomial approximation was obtained (Fig. 5b). The trendline equation is as follows:

where AI is the area index, %, and C is the concentration of the conditioned medium, µl/ml
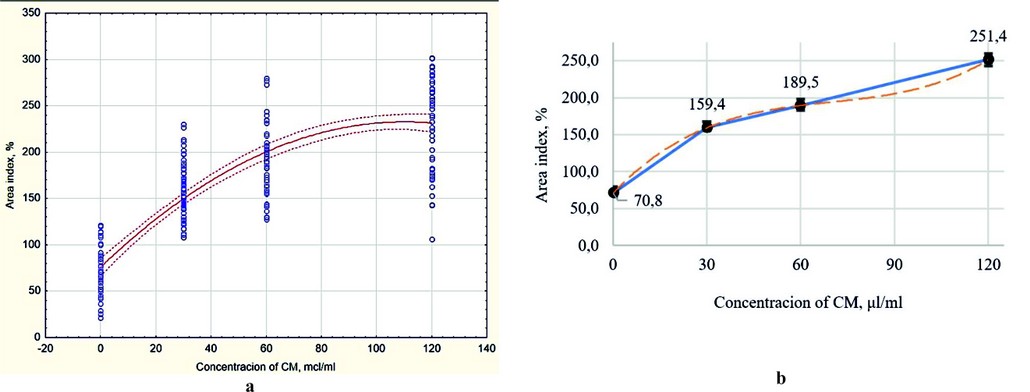
Figure 5. The intensity of the formation of the zone of exclusion and growth of the liver organotypic (organ) culture under the impact of various concentrations of xenogenic MSC secretome (whole conditioned medium): (a) Scatterplot with polynomial fit of results (red line), dotted red line – dotted line – CI95; (b) Scatter chart with bars and markers, built on the medians of the samples; error bars – median errors.
The degree of adhesion in all three studied concentrations also exceeded this indicator in the control, but in the case of 120 μl/ml, the differences were not statistically significant.
The established relationships (2) and (3) between the biological effect and the concentration of the whole MSC secretome can be used to predict the effectiveness of other concentrations, which can be used in the pharmaceutical development of hepatoprotection.
The biological effect of different secretome fractions
After confirming the presence and dose dependence of the regenerative effect on liver tissue caused by a whole conditioned medium containing MSC secretome (exometabolites), the task was to study the effect of its fractions at a concentration of 60 μl/ml on regenerative processes using an organotypic (organ) culture model (Fig. 6).
In addition to the whole conditioned medium, three separate fractions of it of different molecular weights obtained by ultrafiltration were taken into the experiment: below 10 kDa, 10–30 kDa, and over 30 kDa. All fractions of MSC secretome had stimulatory activity compared to the control (p ≤ 0.01). The highest intensity of the formation of the zone of exclusion and growth was manifested in the culture, which was administrated with a low molecular weight fraction of the secretome with a weight of below 10 kDa – an excess of control by 3.2 times; the minor activity was shown by the high molecular fraction weighing over 30 kDa (2.3 times higher than the control). The 10–30 kDa fraction had biological activity at the level of the whole conditioned medium. The adhesiveness in the experimental variants was at the same level as the control.
Most of the factors described in the literature that stimulate liver regeneration are classified as cytokines and chemokines with a molecular weight of over 30 kDa. The data obtained show that the low molecular weight components of the secretome (miRNAs and small peptides) can make an even more significant contribution to the recovery processes.
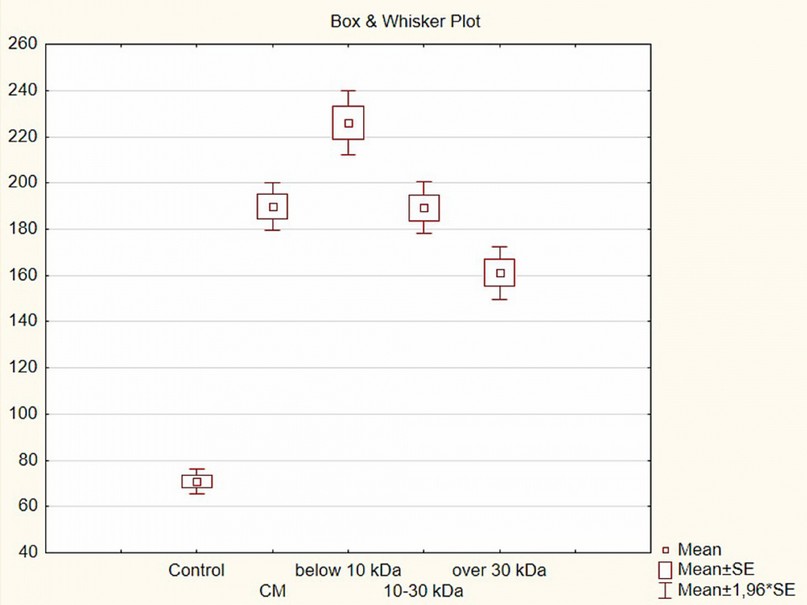
Figure 6. Changes in the formation of the zone of exclusion and growth of the liver organotypic (organ) culture under the impact of various fractions of xenogenic MSC secretome (concentration 60 µl/ml), Box & Whisker Plot
The biological effect of secretome low molecular fraction
At the third stage, the dose dependence of the observed effects for this fraction was studied after determining the most biologically active fraction of the MSC secretome (a low molecular weight fraction with a weight below 10 kDa) (Fig. 7).
The studied dosages were taken as previously described for the whole conditioned medium - 30, 60 and 120 µl/ml, and a concentration of 15 µl/ml was added; a growth medium that does not contain exometabolites was also taken as control. It was shown that the concentration of 15 µl/ml does not significantly affect the regenerative processes in the liver: the area index is at the control level. The remaining concentrations increased the size of the zone of exclusion and growth of culture by 1.3–4.3 times.
Correlation analysis (Fig. 7a) revealed an even stronger relationship between the concentration of the studied composition and the area index than in the case of the whole secretome (r = 0.89; p ≤ 0.01). By using the STATISTICA program, the following equation was obtained that describes the studied dependence:

where AI is the area index, %, and C is the concentration of the below 10 kDa secretome fraction, µl/ml
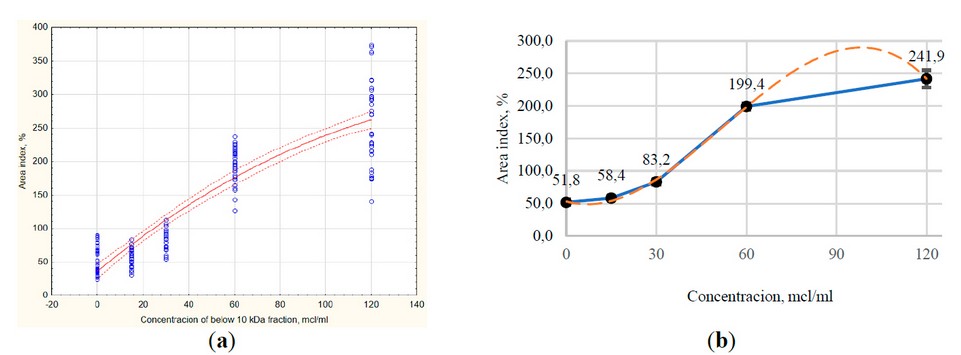
Figure 7. The intensity of the formation of the zone of exclusion and growth of the liver organotypic (organ) culture under the impact of various concentrations of low molecular weight (<10 kDa) fraction of xenogenic MSC secretome: (a) Scatterplot with polynomial fit of results (red line), dotted red line – CI95; (b) Scatter chart with bars and markers, built on the medians of the samples; error bars – median errors.
With the help of a graph built in Excel based on the medians of the samples, a trend line with a polynomial approximation was obtained (Fig. 7b). The trendline equation is as follows:

Where AI is the area index, %, and C is the concentration of the below 10 kDa secretome fraction, µl/ml
The degree of adhesion statistically significantly exceeded the control value only at the maximum concentration of the test substances – 120 µl/ml (p ≤ 0.01).
The established relationships (4) and (5) between the biological effect and the concentration of the low weight fraction of MSC secretome can be used to predict the effectiveness of other concentrations, which can be used in the pharmaceutical development of hepatoprotection. To test the predictive value of equation (5), an additional study of activity at a concentration of 90 μl/ml is necessary (based on the dose-response plot, the effect of this concentration should exceed the effect of a concentration of 120 μl/ml).
DISCUSSION
The samples’ spectrophotometric study determined the protein concentration in them at the level of 3.0-5.5 mg/ml, and nucleic acids – about 2-3.5%. Differences in the absorption spectra of different fractions were shown; they can be used to identify the fraction quickly.
The secretome of MSCs isolated from human bone marrow or adipose tissue has been found to contain from 200 to 600 different proteins. A significant part of the detected proteins (over 75%) were identified as classically secreted proteins by the N-terminal signal peptide 20–24. The described secretome proteins have a wide range of molecular weight – from 10 to 260 kDa, and are at a concentration of about 200.0 μg/ml (about 80 μg/million cells). Over 60% of the protein content is present in the vesicular fraction. Regarding mRNA, it was found that their size does not exceed 31 nucleotides, corresponding to a molecular weight of about 10 kDa 20.
The protein concentration determined by the D280/D260 method (as more sensitive than the V. F. Kalb, R. W. Bernlohr method under these conditions) based on the obtained absorption spectrum was about 3.0-5.5 mg/ml protein (about 500-1000 µg /million cells), which is an order of magnitude higher than in Mitchell et al. 20. Probably, such distortions are associated with the presence of serum albumin in the culture medium (2%) and nutrient medium chromatophores (amino acids and vitamins). To improve the accuracy of spectrophotometric determination of protein concentration in various MSC secretome fractions in this system, empirically established conversion factors that consider the described factors can be used. It is also possible to use serum-free media and purify the components of the medium for the accumulation of exometabolites, but this will lead to a significant increase in the cost of products developed based on this technology.
Chronic liver damage causes liver fibrosis and can lead to end-stage – cirrhosis of the liver. The most effective treatment is liver transplantation from a healthy donor, but this is not always feasible. Therapy of hepatopathy using living MSCs (isolated from bone marrow, adipose tissue, dental pulp, etc. sources) or their exometabolites is discussed as an alternative treatment method. In addition to the ability of MSCs to differentiate themselves into hepatocyte-like cells (this factor is not paramount because they cannot replace all damaged cells due to their relatively small amount), the substances they produce have antifibrotic and immunomodulatory effects, as well as activate the patient’s stem cells, stimulating them homing, division and differentiation. Cell-free therapy based on MSC secretome seems more promising because it does not carry the risks of using living cells – reactive immunogenicity, malignancy, and atypical differentiation. Also, secretome components can be applied to nanoparticles to increase the duration of their circulation in the blood 8, 25–27.
The molecular mechanisms of biological effects of the MSCs secretome are being actively studied. It has been shown that VEGF, HGF, IGF, FGF, TGF-β, Nrf2, HIF, HO-1, IL-6 are responsible for antiapoptotic activity; HO-1 for antioxidant activity; CCL2 (regulation of immunoglobulin synthesis) HGF, TGF-β, IDO, PGE2, IL-10 (regulation of T-lymphocyte proliferation, increasing M2 macrophage polarization, reducing inflammation), PGE2, IL-6, TGF-β, IDO, NO (decreasing natural killer activity) for immunomodulatory activity; and VEGF, HGF, FGF-2, SDF-1, TGF-β, PDGF, ANG1, MCP-1 for angiogenesis 28. It is also known that certain miRNA types exhibit their unique biological effects. For example, miR-199a increases cardiomyocyte proliferation in silico, while miR-126-3p blocks the development of metastases in vivo and in vitro 9.
Studies have shown a positive effect of MSC secretome components on the recovery of damaged liver. Such effects as immunomodulatory mediated by IL-10, HGF, TGF-b3, IDO and PGE2; antifibrotic associated with decreasing the production of extracellular matrix, the amount of alpha-smooth muscle-actin-(a-SMA)-positive liver stellate cells and the activity of pro-fibrogenic genes; and regenerative provided, in particular, by increasing the expression of the genes of oncostatin M, adrenergic receptor 1 and stem cell factor ones were described 10. As was found in the Huh7 cell culture, the components of the conditioned medium containing exometabolites of MSCs significantly change the expression of about 3000 liver cell genes that are responsible for the regulation of the cell cycle, molecular processes with DNA, apoptosis, cell and tissue growth, metabolism, etc. 29. Extracellular secretome vesicles can impact on the liver in two ways: either inhibiting TGF-β1/Smad, Wnt/β-catenin signaling pathways, activating hepatic stellate cells, hepatocyte apoptosis, secretion of inflammatory factors, collagen deposition, or activating autophagy and hepatocyte proliferation 30,31.
The following vital molecules provide a shift in dynamic balance from fibrosis to regeneration: GAS6 activates the healing of damage, complement protein C3 and quiescin-sulfhydryl oxidase 1 stimulates the proliferation of hepatocytes, vitronectin regulates cell adhesion and mobility, matrix metalloproteinase MMP2 cleavage of collagen filaments, protein milk fat globule EGF factor 8 (MFGE8) suppresses the TGF-β signaling pathway and reduces extracellular matrix deposits 32,33. Another study 34 shows high levels of the anti-inflammatory protein Annexin-A1 and suggests a role in stimulating liver repair.
Increasing activity to repair a damaged liver can be achieved in various ways. The most classic example of such an effect is hypoxia during the growth of MSCs. As written by Lee, S. C. et al., the optimal partial pressure of oxygen in culture to obtain a secretome with maximum efficiency is 1% pO2 35. Another way to increase the biological activity of MSC exometabolites may be the administration of lipopolysaccharide into the culture medium 36. The use of MSCs of xenogeneic origin in research and therapy is justified and can be studied 37.
The main biological effects of the MSC secretome, which cause a shift in the dynamic balance of liver processes from fibrotic to regenerative, are the following: inhibition of the TGF-β1/Smad signaling pathway, Wnt/β-catenin pathway, liver stellate cell activity, hepatocyte apoptosis, secretion of inflammatory factors, collagen deposition; activation of autophagy and hepatocyte proliferation 35,10.
The organotypic culture model used in the research allows us to study the effect of secretome components on all populations of liver cells. Even though this express model does not give an idea of which cells colonize the space around the explant, the newly formed zone’s area indicates the tissue’s regenerative potential. Fibrotic processes can close holes and gaps but cannot underlie the settlement of new areas.
The data obtained do not contradict the data of literary sources regarding the regenerative ability of the components of the secretome of mesenchymal stem cells but allow us to expand our understanding of the possible mechanisms of the observed effect. It was shown that the low molecular weight fraction of the secretome with a mass of up to 10 kDa has the highest biological activity, which indicates a significant contribution of miRNAs and low molecular weight peptides that are not related to growth factors and cytokines to the start of liver tissue repair.
CONCLUSIONS
The study of the component composition of the secretome of MSCs is carried out using various methods of analysis: mass spectrometry to characterize the spectrum of proteins, HPLC to separate and identify individual components, ELISA to accurately determine the qualitative and quantitative composition of proteins, and microRNA chips to study RNA molecules. The spectrophotometric analysis of the secretome composition used in this study must be more accurate. However, it provides high reproducibility in small laboratories and can also be used in routine quality control in production or screening studies.
Spectrophotometric study of the samples determined the concentration of proteins in them at the level of 3.0-5.5 mg/ml. The absorption spectra of fractions separated by mass do not contain a pronounced peak at 260 nm, characteristic of nucleic acids and several proteins. At the same time, peaks are observed in the low molecular weight fractions at 220 nm (sulfur-containing amino acids), 240 and 280 nm (aromatic amino acids), and 340 nm (complex peptides, possibly with metal domains). The high molecular weight fraction of the secretome contains a bandwidth of 280 nm, which indicates the presence of complex proteins containing, in particular, a significant amount of aromatic amino acids.
It was found that secretome containing exometabolites of xenogenic mesenchymal stem cells stimulates liver regeneration in vitro. The concentration of secretome components and their biological effect have a significant and robust positive correlation, which makes it possible to predict the effect of unstudied concentrations on the liver by using the equations obtained. The fraction of MSC secretome with a molecular weight below 10 kDa had the most significant effect. This fraction does not contain the most studied cytokines and chemokines. Low molecular weight miRNAs and peptides likely play an essential role in triggering liver regeneration processes.
A significant strong correlation was shown between the concentration of exometabolites with a molecular weight below 10 kDa and the observed biological activity, which makes it possible to predict the effect of other concentrations of this fraction on liver recovery. These data can be used in the pharmaceutical development of hepatoprotection
Funding: This research received no external funding.
Institutional Review Board Statement: All manipulations with animals complied with international legislation requirements for the protection of vertebrate animals 29, and the Bioethics Commission approved the study. The study protocol and report were approved at meetings of the Bioethical Commission of the Department of Molecular Biology and Biotechnology of the Faculty of Biology of V.N. Karazin Kharkiv National University (Protocol No. 1, 2021/08/27).
Acknowledgments: The author expresses his gratitude to Alexey Lavrik for the idea for the study, Oksana Novikova and Anatoly Goltvyansky for help in developing the methods and providing reagents, scientific adviser Professor Anatoly Bozhkov for critical remarks in the planning and implementation of the study, preparation of the manuscript, as well as for the opportunity to work in the laboratory and perform research.
Conflicts of Interest: The author declares no conflict of interest.
REFERENCES
1. Asrani, SK; Devarbhavi, H.; Eaton, J.; Kamath, P.S. Burden of liver diseases in the world. J. Hepatol. 2019, 70(1), pp. 151-171. doi: 10.1016/j.jhep.2018.09.014
2. Bosek, P.; Nakano, M. Hepatoprotective effect of rooibos tea (Aspalathus linearis) on CCl4-induced liver damage in rats. Physiol. Res. 2003, 52(4), pp. 461-466.
3. Rajesh, M.G.; Latha, MS Hepatoprotection by Elephantopus scaber Linn. in CCl4-induced liver injury. Indian J. Physiol. Pharmacol. 2001, 45(4), pp. 481-486.
4. Tedesco, D.; Tava, A.; Galletti, S.; Tameni, M.; Varisco, G.; Costa, A.; Steidler, S. Effects of silymarin, a natural hepatoprotector, in periparturient dairy cows. J. Dairy Sci. 2004, 87(7), pp. 2239-2247.
5. Wang, X.; Yu, H.; Xing, R.; Li, P. Hepatoprotective effect of oyster peptide on alcohol-induced liver disease in mice. Int. J. Mol. Sci. 2022, 23(15), p. 8081. doi: 10.3390/ijms23158081
6. Cho, H.R.; Lee, S.O. Novel hepatoprotective peptides derived from protein hydrolysates of mealworm (Tenebrio molitor). Food Res. Int. 2020, 133, p. 109194. doi: 10.1016/j.foodres.2020.109194
7. Mora, S.I.; García-Román, J.; Gómez-Ñañez, I.; García-Román, R. Chronic liver diseases and the potential use of S-adenosyl-L-methionine as a hepatoprotector. Eur. J. Gastroenterol. Hepatol. 2018, 30(8), pp. 893-900. doi: 10.1097/MEG.0000000000001141
8. Hu, C.: Zhao, L.; Zhang, L.; Bao, Q.; Li, L. Mesenchymal stem cell-based cell-free strategies: safe and effective treatments for liver injury. Stem Cell Res. Ther. 2020, 11(1), pp. 1-12. doi:10.1186/s13287-020-01895-1
9. Eleuteri, S.; Fierabracci, A. Insights into the secretome of mesenchymal stem cells and its potential applications. Int. J. Mol. Sci. 2019, 20(18), p. 4597. doi:10.3390/ijms20184597
10. Driscoll, J.; Patel, T. The mesenchymal stem cell secretome is an acellular regenerative therapy for liver disease. J. Gastroenterol. 2019, 54(9), pp. 763-773. doi:10.1007/s00535-019-01599-1
11. Harrell, C.R.; Fellabaum, C.; Jovicic, N.; Djonov, V.; Arsenijevic, N.; Volarevic, V. Molecular mechanisms responsible for therapeutic potential of mesenchymal stem cell-derived secretome. Cells. 2019, 8(5), p. 467. doi:10.3390/cells8050467
12. Gnecchi, M.; Danieli, P.; Malpasso, G.; Ciuffreda, MC Paracrine mechanisms of mesenchymal stem cells in tissue repair. Mesench. stem cells. 2016, pp. 123-146. doi:10.1007/978-1-4939-3584-0_7
13. Colter, D.C.; Class, R.; DiGirolamo, C.M.; Prockop, D.J. Rapid expansion of recycling stem cells in cultures of plastic-adherent cells from human bone marrow. Proc. Natl. Acad. Sci. 2000, 97(7), 3213-3218. doi: 10.1073/pnas.97.7.3213
14. Freshney, R.I.; Capes-Davis, A.; Gregory, C.; Przyborski, S. Culture of animal cells: a manual of basic technique and specialized applications, 7th ed.; John Wiley & Sons, Inc.: Hoboken, New Jersey, USA, 2016.
15. Ogurtsov, A.N.; Bliznyuk, O.N.; Antropova, L.A. Fiziko-khimichtskiie osnovy biotekhnologii: praktikum [Physical and chemical foundations of biotechnology: tutorial]; Vydavnytstvo NTU "KhPI", Kharkov, Ukraine, 2014. (in Russ.).
16. Khavinson, V.H.; Chalisova, N.I.; Linkova, N.S.; Khalimov, R.I.; Nichik, T. E. Zavisimost tkaniespiecyfichieskogo diejstviia peptides ot kolichiestva aminokislot, vkhodiaschikh v ikh sostav [The dependence of tissue-specific peptides activity on the number of amino acids in the peptides]. Fundament. Res. 2015, (2-3), pp. 497-503. (in Russ.).
17. Atramentova, L.A.; Utevskaia, O.M. Statisticheskie metody v biologii uchebnik [Statistical methods in biology: textbook]. Vydavnytstvo Likhtar: Gorlovka, Ukraine, 2008. (in Russ.).
18. Lapach, S.N.; Chubenko A.V.; & Babich P.N. Statisticheskie metody v mediko-biologicheskikh issledovaniiakh s ispolzovaniem Excel [Statistical methods in biomedical research using Excel], 2nd ed.; Morion: Kiev, Ukraine, 2001. (in Russ.).
19. European Commission. Council Directive 86/609/EEC of 24 November 1986 on the approximation of laws, regulations and administrative provisions of the Member States regarding the protection of animals used for experimental and other scientific purposes. O. J. European Communities. 1986, 358, pp. 1-28.
20. Mitchell, R.; Mellows, B.; Sheard, J.; Antonioli, M.; Kretz, O.; Chambers, D., Zeuner, M.-T., Tomkins, J. E., Denecke, B., Musante, L., Joch, B., Debacq-Chainiaux, F., Holthofer, H., Ray, S., Huber, T. B., Dengjel, J.; de Coppi, P.; Widera, D.; Patel, K. Secretome of adipose-derived mesenchymal stem cells promotes skeletal muscle regeneration through synergistic action of extracellular vesicle cargo and soluble proteins. Stem Cell Res. Ther. 2019, 10(1), pp. 1-19. doi:10.1186/s13287-019-1213-1
21. Ragni, E.; Orfei, C.P.; de Luca, P.; Mondadori, C.; Viganò, M.; Colombini, A.; de Girolamo, L. Inflammatory priming enhances mesenchymal stromal cell secretome potential as a clinical product for regenerative medicine approaches through secreted factors and EV-miRNAs: the example of joint disease. Stem Cell Res. Ther. 2020, 11(1), pp. 1-19. doi:10.1186/s13287-020-01677-9
22. Kalinina, N.; Kharlampieva, D.; Loguinova, M.; Butenko, I.; Pobeguts, O.; Efimenko, A.; Ageeva, L.; Sharonov, G.; Ischenko, D.; Alekseev, D.; Grigorieva, O.; Sysoeva, V.; Rubina, K.; Lazarev, V.; Govorun, V. Characterization of secretomes provides evidence for adipose-derived mesenchymal stromal cells subtypes. Stem Cell Res. Ther. 2015, 6(1), pp. 1-12. doi:10.1186/s13287-015-0209-8
23. Riis, S.; Stensballe, A.; Emmersen, J.; Pennisi, C.P.; Birkelund, S.; Zachar, V.; Fink, T. Mass spectrometry analysis of adipose-derived stem cells reveals a significant effect of hypoxia on pathways regulating extracellular matrix. Stem Cell Res. Ther. 2016, 7(1), pp. 1-14. doi:10.1186/s13287-016-0310-7
24. Baberg, F.; Geyh, S.; Waldera-Lupa, D.; Stefanski, A.; Zilkens, C.; Haas, R.; Schroeder, T.; Stühler, K. Secretome analysis of human bone marrow derived mesenchymal stromal cells. Biochim. Biophys. Acta Proteins Proteom. 2019, 1867(4), pp. 434-441. doi:10.1016/j.bbapap.2019.01.013
25. Nazarie, S.R.; Gharbia, S.; Hermenean, A.; Dinescu, S.; Costache, M. Regenerative potential of mesenchymal stem cells’(MSCs) secretome for liver fibrosis therapies. Int. J. Mol. Sci. 2021, 22(24), 13292. doi:10.3390/ijms222413292
26. El Moshy, S.; Radwan, I.A.; Rady, D.; Abbass, M.; El-Rashidy, A.A., Sadek, K.M.; Dörfer, Ch.E.; Fawzy El-Sayed, K.M. Dental stem cell-derived secretome/conditioned medium: the future for regenerative therapeutic applications. Stem Cells Int. 2020, 2020. doi:10.1155/2020/7593402
27. Liang, H.; Huang, K.; Su, T.; Li, Z.; Hu, S.; Dinh, P.U.; Wrona, E.A.; Shao, C.; Qiao, L.; Vandergriff, A.C.; Hensley, M.T.; Cores, J.; Allen, T.; Zhang, H.; Zeng, Q.; Xing, J.; Freytes, D.O.; Shen, D.; Yu, Z.; Cheng, K. Mesenchymal stem cell/red blood cell-inspired nanoparticle therapy in mice with carbon tetrachloride-induced acute liver failure. ACS Nano. 2018, 12(7), 6536-6544. doi:10.1021/acsnano.8b00553
28. González-González, A.; García-Sánchez, D.; Dotta, M.; Rodríguez-Rey, J.C.; Pérez-Campo, F.M. Mesenchymal stem cells secretome: The cornerstone of cell-free regenerative medicine. World J. Stem Cells. 2020, 12(12), p. 1529. doi:10.4252/wjsc.v12.i12.1529
29. Fouraschen, S.M.; Pan, Q.; de Ruiter, P.E.; Farid, W.R.; Kazemier, G.; Kwekkeboom, J.; Ijzermans, J.N.M.; Metselaar, H.J.; Tilanus, H.W.; de Jonge, J.; van der Laan, LJ Secreted factors of human liver-derived mesenchymal stem cells promote liver regeneration early after partial hepatectomy. Stem Cells Dev. 2012, 21(13), pp. 2410-2419. doi:10.1089/scd.2011.0560
30. Gharbia, S.; Hermenean, A.; Dinescu, S.; Costache, M. Regenerative potential of mesenchymal stem cells’(MSCs) secretome for liver fibrosis therapies. Int. J. Mol. Sci. 2021, 22(24), p. 13292. doi:10.3390/ijms222413292
31. Jun, J.H.; Kim, J.Y.; Choi, J.H.; Lim, J.Y.; Kim, K.; Kim, G.J. Exosomes from placenta-derived mesenchymal stem cells are involved in liver regeneration in hepatic failure induced by bile duct ligation. Stem cells int. 2020, 2020 doi:10.1155/2020/5485738
32. Kim, D.; Cho, G.S.; Han, C.; Park, D.H.; Park, H.K.; Woo, D.H.; Kim, J.H. Current understanding of stem cell and secretome therapies in liver diseases. Tissue Eng. Regen. Med. 2017, 14(6), 653-665. doi:10.1007/s13770-017-0093-7
33. An, S.Y.; Jang, Y.J.; Lim, H.J.; Han, J.; Lee, J.; Lee, G.; Park, J.Yo.; Park, S.-Yo.; Kim, J.H.; Do, B.-R.; Han, Ch.; Park, H.-K.; Kim, O.H.; Song, M.J., Kim, S.-J.; Kim, J.H. Milk fat globule-EGF factor 8, secreted by mesenchymal stem cells, protects against liver fibrosis in mice. Gastroenterology. 2017, 152(5), pp. 1174-1186. doi:10.1053/j.gastro.2016.12.003
34. Zagoura, D.; Trohatou, O.; Makridakis, M.; Kollia, A.; Kokla, N.; Mokou, M.; Psaraki, A.; Eliopoulos, A.G.; Vlahou, A.; Roubelakis, M.G. Functional secretome analysis reveals Annexin-A1 as important paracrine factor derived from fetal mesenchymal stem cells in hepatic regeneration. EBioMedicine. 2019, 45, pp. 542-552. doi:10.1016/j.ebiom.2019.07.009
35. Lee, S.C.; Kim, K.H.; Kim, O.H.; Lee, S.K.; Hong, H.E.; Won, SS; Jeon, S.-J.; Choi, B.J.; Jeong, W.; Kim, S.J. Determination of optimized oxygen partial pressure to maximize the liver regenerative potential of the secretome obtained from adipose-derived stem cells. Stem Cell Res. Ther. 2017, 8(1), pp. 1-12. doi:10.1186/s13287-017-0635-x
36. Lee, S.C.; Jeong, H.J.; Lee, S.K.; Kim, S.J. Lipopolysaccharide preconditioning of adipose-derived stem cells improves liver-regenerating activity of the secretome. Stem Cell Res. Ther. 2015, 6(1), pp. 1-11. doi:10.1186/s13287-015-0072-7
37. Li, T.; Yan, Y.; Wang, B.; Qian, H.; Zhang, X.; Shen, L.; Wang, M.; Zh, W.; Li, W.; Xu, W. Exosomes derived from human umbilical cord mesenchymal stem cells alleviate liver fibrosis. Stem Cells Dev. 2013, 22(6), p. 845. doi:10.1089/scd.2012.0395
Received: 25 June 2023/ Accepted: 26 August 2023 / Published:15 September 2023
Citation: Moskalov, V. Effects of various xenogenic mesenchymal stem cell secretome fractions on the regenerative capacity of the liver in vitro. Revis Bionatura 2023;8 (3) 90 http://dx.doi.org/10.21931/RB/2023.08.03.90