CS 2019.02.01.23
Files > Conference Series > 2019 > Humboldt Kolleg 2019
Bionatura Conference Series Vol 2. No 1. 2019
“Breaking Paradigms: Towards a Multi-, Inter- and Transdisciplinary Science” In commemoration of the 250th Anniversary of Alexander von Humboldt
Gene Therapy: An Outstanding Technique For Diseases
Nayade Sarmiento, Selena Tinoco
available in: http://dx.doi.org/10.21931/RB/CS/2019.02.01.23
ABSTRACT
Nearly three decades ago, the first clinical trial based on gene therapy revolutionized the scientific field by demonstrating that it was possible to genetically modify harmful or defective cells and therapeutically improve patient conditions. Since then, this technique has been implemented for the study and treatment of different diseases, many of them fatal, with the hope of achieving a "cure". Several genetic editing tools have been developed and continue to be studied to improve the effectiveness of gene therapy, in addition to a more exhaustive analysis for choosing the type of vector, which is the main cause of adverse effects. In this review, we present characteristics of the gene therapy mechanism along with the types of vectors that are used in this procedure, followed by the most important applications in the medical field and briefly we describe some limitations and prospects to study in the future.
Keywords: gene therapy, vector, adverse effects
INTRODUCTION
Gene therapy is defined as the procedure capable of treating acquired or inherited diseases by genetically modifying the patient's cells by inserting genetic material through vehicular vectors within the nucleus of the target cell, correcting the loss of function caused by different mutations1. Mainly, what this technique does is replace the malfunctioning genes that generate diseases by "good" genes2. Gene therapy is divided into two groups or categories: germline gene therapy, based mainly on the passage of the therapeutic gene (gene transfer) to daughter cells (next generation); and somatic line gene therapy based on the insertion of the therapeutic gene in the target cells but without passing the change to the next generation3.
The first clinical study based on gene therapy was conducted in 1989 by the National Institutes of Health (NIH), which focused on genetically modifying lymphocytes from tumors through retroviral vectors, with the aim of locating this type of cells in the patient as they were reinfused4,5. This approach demonstrated that cells could be genetically modified and reinserted into patients without pain4. However, depending on the type of vector that is used for the gene transfer, this process can be therapeutically successful or present adverse effects, so scientists have carried out different studies to develop and improve the efficiency of the vectors in order to reduce off-target effects6. There are four fundamental steps to perform gene therapy: first, you must identify the mutated gene that is causing the disease; second, the identical healthy gene is cloned or also called the therapeutic gene or transgene; third, the transgene is introduced into a vector that will help transfer the desired gene to the target cell of the patient and fourth, once the vector is inside the target cell, it will release its genetic material by integrating to the cell's DNA, thus correcting the defective gene1.
To date, around 2600 clinical trials have been performed worldwide using gene therapy to combat diseases such as cancer, using Chimeric Antigen Receptors (CARs) in T cells to treat B-cell tumors; monogenic diseases for the treatment of cystic fibrosis, monogenic mitochondria disorders and disorders belonging to bone marrow; infectious diseases based on HIV and cardiovascular diseases on ischaemia, all with high mortality rates4. However, there are still several challenges to overcome in order to achieve a high level of efficacy in this type of procedure, since inserting a therapeutic gene into the patient's body does not always result in the removal of the mutated gene or in the desired effects, for what developing more precise genetic editing techniques such as ZFN, TALENs and CRISPR-Cas9 allow to improve gene therapy and its limitations2. In this paper, we present a review of the gene therapy technique together with the type of vectors that are used, the genetic editing tools implemented to improve its procedure and its applications in the different fields of the medical area, besides mentioning its limitations and future prospects, in order to have a better knowledge about this powerful tool that could be able to “cure” several diseases.
GENE DELIVERY
Delivery techniques in gene therapy are very important because of its purpose to successfully carry the desired gene to target cells of the patient's body, through the use of vectors as vehicles6. These vectors are classified mainly into two types: viral and non-viral vectors.
Viral vectors
Viral vectors come mainly from the viruses that infect mammals7 the most prominent being: gamma-trovirus, adenovirus, lentivirus, herpes simplex virus and adeno-associated virus (AAV)6. The use of this type of virus allows the proteins found on the surface of the viral particle to interact with the receptor of the target cell due to transduction (transfer of hereditary traits to other bacteria strains3, which produces endocytosis and once it is inside the cell, the virus releases the virus genetic information (with the desired gene) to the nucleus so it can be expresse7. Gammaretrovirus and lentivirus are both retroviruses, which by means of the transduction process, insert the DNA or its genome inside the infected cell6 and when this happens, the provirus will be passed onto the cell daughters of the cells that have been infected5. Unlike the gammaretrovirus that can only transduce proliferating cells, lentiviruses can transduce proliferating and non-proliferating cells, which causes a longer expression of the gene, making them better vectors than gammaretrovirus for use in gene therapy. One problem with the use of retroviruses is that, by inserting the genome of a virus into the host cell, insertional mutagenesis can be caused, which means that the genome was placed in an incorrect location causing the disruption of the tumors suppression genes, resulting in the activation of oncogenesis7.
In the case of adenovirus, they are considered the most used vectors in clinical studies representing 20.5% of all trials4 because of its high transduction efficiency in gene delivery to replicating and non-replicating cells and high levels of gene expression6. Because adenoviruses possess their genome as an episome in the host nucleus, this prevents a mutagenic insertion compared to retroviruses. Its main function is in oncolysis when killing and infecting tumor cells since when it is inserted into the tumor mass, it activates the antitumor immunity causing the death of the tumor7.
The adeno-associated virus (AAV) also transduces dividing cells and non-dividing cells and in the same way as the adenoviral vectors, their genome remains episonally in the nucleus of the host. Its application focuses on the central nervous system (CNS) by being able to cross the barriers of the brain and target and transduce cells of CNS7. The main limitation with this type of vector is that it can only transduct a small amount of DNA (<5kb) so it cannot deliver large therapeutic genes6. Herpes Simplex Virus (HSV) has its application in neurological disorders since when applying this vector in the patient's body, it is taken by the central nerve allowing it to travel through the neurons until reaching the neuronal cell body and releasing its genome inside the nucleus7. This type of vector can deliver large amounts of DNA (-40kb) in addition to being able to infect non-dividing cells6.
Non-viral vectors
On the other hand, non-viral vectors or DNA vectors are those that use a "naked" DNA to be administered in different tumor and non-tumor tissues4, which means that it is not combined with the genome of the host resulting in its lost once there is cell division6. The vector most used for its easy and simple handling in addition to large scale production is Plasmid DNA, which is inserted into the target cell as an episome generating significant levels of gene expression4. In order to improve the efficiency of delivery and gene expression of the non-viral vectors, physical methods have been implemented such as incorporating Plasmid DNA into liposomes or nanoparticles, or the use of electroporation or gene gun to achieve better delivery of the gene of interest towards the target cell6,7.
GENOME EDITING TOOLS
Gene mutation
Considered as a simple way to perform genetic editing, the gene knockout takes advantage of the errors in the NHEJ, non-homologous end joining, to add indels to a specific site. Commonly this system re-links two edges of DNA without any revision, this is why the modified system, microhomology-mediated end joining, adds micro-homologous regions that after annealing joins with the DNA. Both processes have significant mutagenesis rates giving way to the formation of indels at the cutting site. These generated indels can cause changes in the reading frame of a gene, allowing the formation of many diseases. However, this disadvantage of mutagenesis is often used to induce mutations and eliminate a gene of interest2.
Gene edition
Another way to delete DNA fragments is to use a double strand break, DSB. By causing two simultaneous breaks larger deletions could be obtained, which is beneficial in cases where it is necessary to eliminate complete or large genomic segments. In the case of gene correction, one option is to use specific DSBs in which HDR is used in conjunction with the desired fragment, which would have the role of a repair template. If there is a difference between the template and the sequence to be modified, these differences are easily incorporated, allowing the correction of diseases linked to a mutation. Some of the DNA donors that are available for this method are single-stranded oligonucleotides, viral vectors, and adeno-associated viruses. One option for gene insertion is to edit the genome by means of a double strand break induced by nucleases, in this way compatibility between the target DNA and the template DNA will be obtained. This would lead to a NHEJ mediated by ligation of both the DNA that is inserted and the target DNA2.
Targeted nucleases
Genome editing using ZFN, zinc finger nuclease, starts when a DSB is made removing a specific DNA from a site of interest. Then the NHEJ or HDR repair methods will be employed. In this way, the NHEJ repair system can be used to perform insertions or deletions against a target gene9. Secondly, TALENs, transcription activator-like effector nucleases, are those nucleases that aim to edit the genome more effectively than previously used nucleases. When referring to TALENs, we refer to nucleases that join a target sequence in a specific site and then cause a rupture in a double chain. This rupture will be remedied by processes such as HDR or NHEJ8. The CRISPR-Cas9 system, clustered regularly interspaced short palindromic repeats, associated Cas9 nuclease, is based on the bacteria's immune system and its primary function, which is to protect bacteria from any invader. The process implemented in gene therapy consists of modifying the guide RNA, which will have the target DNA sequence that will be recognized by the Cas9 endonuclease, then Cas9 nuclease will look for this target sequence in the genome of the organism to completely eliminate it10. This system has been selected to attack specific sequences of target DNA in numerous clinical trials, demonstrating a lot of efficiency and precision in attacking the desired DNA while reducing the risks of side effects for the patient4.
APPLICATIONS
Several clinical trials based on gene therapy have been applied more frequently in the last two decades, although only some of them have had positive and effective results while others have presented adverse effects due to the type of vector that was used in the patients11. To date, there are 4 outstanding applications: the first place is for the treatment of cancer (66,6% of clinical trials), the second place in monogenic diseases (11.5%) with the highest rate of effectiveness, the third is in infectious diseases (6,3%) and fourth in cardiovascular diseases (6.2%)12 (Figure 1).
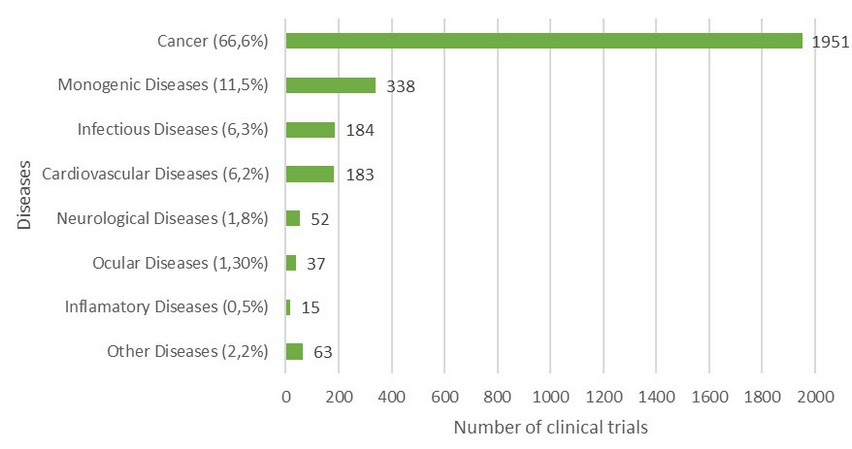
Figure1. Gene Therapy Clinical Trials Worldwide. The graph shows number of clinical trials per disease, performed until December 2018. Adapted from Reference 12.
Cancer
Cancer represents one of the most serious and difficult diseases for treatment and by using gene therapy as a solution, it has opened a large field of research. Currently, several types of cancer have already been studied in clinical trials such as lung, skin, neurological, urological, gastrointestinal and leukemia cancer4. The treatment for cancer immunotherapy is considered one of the most important applications of gene therapy because it has had an impressive development in the medical area2. Mainly, the T cells (cells of the immune system) are used in this type of therapies since by genetically modifying them they can attack specific cancer antigens ex vivo and then infuse it back into the patient11, being successful in cases of leukemia, lymphoma, and melanoma2.
The most recent application is based on the introduction of chimeric antigen receptors (CARs) in T cells. CARs are chimeric because they have parts of different antibodies that when it is bound to a T cell, it creates a modified T cell (CAR T cell) with the ability to recognize specific antigens (epitopes) in cancer cells in vivo11. The most recent treatment with CAR T cells was to transfer a gene of interest to a CAR that recognizes a B-cell surface marker CD19 to T cells ex vivo, and what was found was that the engineered T cells anti-CD19 had the ability to reverse the advanced stage of lymphoma in several patients13 reducing its toxicity and making it much more manageable11. This approach has shown great importance and effectiveness for what is already at the time several clinical trials studying its application in malignant tumors of B cells5.
Monogenic diseases
A monogenic disease occurs when a gene undergoes modifications affecting all the existing cells in the human body14. According to their genetic origin, monogenic diseases can be classified as dominant, recessive and X-linked. Within each pair of chromosomes, humans possess a copy of each gene, that is, they have two copies of the same gene, allele. Dominant monogenic diseases affect copies of individual genes, whereas recessive monogenic diseases focus on directly damaging copies and alleles15. On the other hand, monogenic diseases linked to the X chromosome are those related to genes that affect said chromosome. This type of diseases linked to X chromosome tends to have a greater incidence in men than in women because men only have a copy of the X chromosome16.
An erroneous thought that has been distributed in society is that this type of disorder is unusual, however until 2016, more than five thousand different monogenic diseases were found14. Recently, it has been shown that there are millions of cases around the world of people affected by this condition. While the type of disorder attacks a significant number of lives worldwide, its true magnitude is significant when taking into account the damages caused by its most representative diseases such as cystic fibrosis, sickle cell anemia, hemophilia A and B, etc.15. Some of the conditions that these diseases cause are immunodeficiency, also known as dysregulation17, specific attacks against lysosomes, hemoglobin, mitochondria, among others18. Since conventional medical treatments have not been sufficient to combat the symptoms of affected patients, techniques have been sought that can successfully counteract the problems generated by this condition. Being one of the most mentioned recently the use of gene therapy18.
The main reason why we seek to use gene therapy to combat these disorders is to directly attack the gene that has undergone a mutation giving way to severe consequences4. In this way, the aim is to correct the gene and subsequently reinsert it into cells that, through its division, help to spread it, this is a measure taken to ensure that the insertion and multiplication of the corrected gene will be successful. However, the use of viral vectors for gene transport is one of the most acclaimed methods, since it has been used in enough clinical trials to ensure its effectiveness. Many monogenic diseases have been treated with genetic editing, here are mentioned some of the most known and what has been their advances over time14.
One of the most well-known diseases that have been treated significantly is cystic fibrosis. This hereditary disease is characterized by being autosomal recessive affecting mainly the gene that regulates the conductance of fibrous cystic transmembrane, CFTR. This gene codes for an anionic epithelial channel, which is present mainly in the digestive and intestinal tract, resulting in a fluid accumulation14. As time goes by, this mutation in the CFTR gene causes other organs such as the pancreas and lungs to be compromised due to thickening of the mucosa. The most usual treatments include antibiotics, physical therapy, and nutritional advice, with them, it has been possible to alleviate the symptoms of the patients but not to cure the disease. Some of the methods used to treat this disease include the use of antiviral and non-viral vectors, however, both have had difficulties that have not been overcome and have been inefficient in the delivery of genetic material. Also, transcription activator-like effector nucleases, TALENs, have been used for genetic editing, and compared with the CRISPR-Cas9 system, they can carry longer sequences ensuring a high degree of efficacy19.
The disorders of monogenic mitochondria are not the only ones that affect the mitochondria of the human body, mitochondrial dysfunction is more common than it is thought and it drastically affects the health of a person when it joins with other pathological diseases such as obesity, Alzheimer's, Parkinson, etc. Toxins from the environment are another cause that can interfere with regular mitochondrial function. Many of the mitochondrial proteins are encoded by more than 1000 genes, a change in these genes results in mitochondrial dysfunction. These mutations reach to affect in a great size to different organs causing together a decrease in the amount of available energy in the body, a decrease of ATP. It is for this reason that effective and simple solutions are sought to combat the effects caused by this disease14.
Since 2006, different clinical trials have been conducted in which vitamins were included in order to cure the disease, although no reliable or effective results were obtained. Different clinical trials have conducted tests with the drug idebenone, which is a variant of CoQ10, has also been thought of the application of a ketogenic diet due to its contribution in the reduction of epileptic seizures in patients who showed problems of electron transport. Another possibility is to modify the mutations that are generated in the genes coding for mitochondrial proteins, in this way the problem could be solved more directly. Many of the treatments designed to cure this disorder must go through more studies in order to provide competent results18.
The monogenic disorder belonging to the bone marrow is related to a significant number of affections ranging from bone marrow to symptoms of failure syndromes15, including also problems related to immunodeficiency. Blood transfusions of erythrocytes or platelets are made within the existing treatments to fight against this conditions20. One of the methods for the treatment of this disease is the transplantation of hematopoietic stem cells. However, some patients present problems to find an adequate donor, in these cases these patients must receive gene therapy15.
By inserting hematopoietic stem cells into the patient that have been previously corrected, excellent results were expected. However, poor management of the vectors used to reinsert genes that have undergone mutations within the patients' genome, led to many of them developing serious diseases such as leukemia. One of the main reasons for the appearance of this disease is that there is a failure in the insertion mutagenesis. Currently, clinical trials are carried out with improved vectors and attempts are being made to incorporate gene therapy to modify autologous stem cells. Using modern techniques such as CRISPR-Cas, we intend to modify selected sequences so that homologous recombination can insert the correct gene, thus fixing dysfunctional genes. The use of ZFN and TALENs has also been used to combat this condition15.
Infectious Diseases
Up to the year 2017, 182 clinical trials were registered mainly directed towards infectious diseases, representing in first place HIV (69.2% of total trials of infectious diseases), followed by hepatitis B and C (11%), and malaria treatment (7.1%)4. During the investigations, the AAV vector was mostly used to administer genes that encode antibodies for specific epitopes derived from pathogens, obtaining, as a result, an effective vector that produces antibodies specific for pathogens that help to fight and protect the organism from future infectious diseases such as HIV. Clinical studies on HIV applying gene therapy are in the first phase of exploration13.
Cardiovascular diseases
One of the most recent approaches to gene therapy is the treatment of cardiovascular diseases because using conventional pharmacological treatments is almost impossible to eradicate them. Although techniques in this field have not advanced much over the years, some of the areas that could be worked on are therapeutic angiogenesis, repair, and regeneration, improve existing faults in bypass, among others21,22. Gene therapy has shown a high capacity for the modification of genes that reduce cardiac problems, its effectiveness has been proven in tissues both in vitro and in vivo. It is believed that one of the greatest advantages of the application in tissues could be that, the obtained result is maintained in the long term without the presence of negative side effects for the patient. Also, it should be taken into account that more studies are required that allow the efficient delivery of modified genes. Among the objectives to improve is to enhance the viral genes that are responsible for delivering the genetic material properly. The blood vessels are the most studied targets to fight this type of diseases because they are easy to access, other points of interest are the local improvement of endothelium, liver, myocardium, and arteries23. One of the recently mentioned methods in the treatment of cardiovascular diseases is the CRISPR-Cas, being the most known areas of interest in the cardiac system and the vascular system24. There are specific points that can be easily targeted and that will surely be more studied in the future 22 (Figure 2).
One of the common cardiovascular diseases is ischemia, which occurs due to lack of oxygen. This disease is divided into two types: myocardial ischemia, produced by coronary artery disease, and lower limb ischemia, produced by peripheral artery disease. One solution that has called attention to the treatment of this disease is the use of therapeutic angiogenesis to increase the number of vessels in ischaemic tissue4. For this, growth factors of angiogenesis such as vascular endothelial growth factor, VEGF, of type A, B, C, D, and E. The use of these growth factors has given good results in tests performed on ischaemic extremities of rabbit and in animals for experiments whose arteries were affected. However, the efficacy and safety of the use of these factors are not completely proven. Therefore, other available alternatives are to employ drug-stimulated angiogenesis or electricity. A local application of a gene in the heart or in blood vessels can also be performed25.
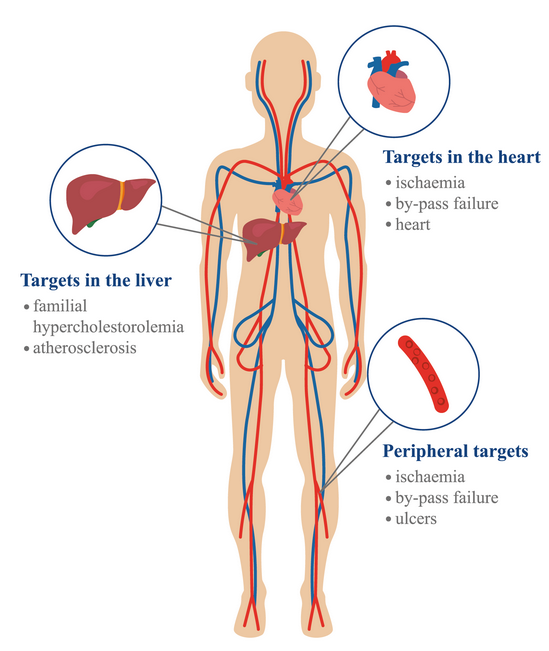
Figure2. Targets for further cardiovascular gene therapy investigation
Future Prospects
Gene therapy has been applied for many years demonstrating high efficiency and improving initial failures in clinical trials. However, there are still many details to improve to ensure effective results in future trials. One of the first aspects that need to be improved is the delivery of genetic material through vectors of different types. One of the greatest risks when using viral vectors is having high rates of insertional mutagenesis and genotoxicity, in addition to the generation of side effects in patients. One of the options that are being considered to improve the efficacy of these vectors is to genetically modify their protein surface, thus reducing the impact of vector entry into the organism. These vectors are being widely used in trials against diseases such as malaria, HIV, and Zika, opening a window to the treatment of these diseases26. Non-viral vectors have certain advantages over viral vectors such as their easy construction, ability to deliver large genes and low immunotoxicity effects. Despite this, improvements are still needed to combat cell-type mechanisms and thus improve their effectiveness7.
Another point to be discussed is that despite the rapid progress in clinical trials for the treatment of malignant tumors affected by B cells using CARs, this also affects the level of B cells in the patient. The lack of these cells can be remedied by infusions of immunoglobulins but other side effects are not so easy, so more studies are needed to continue the use of this technique. Other questions that remain to be resolved are how to reduce the level of toxicity in the process and how to improve the performance of the technique in more complicated cancers such as solid cancers11.
In the field of infectious diseases, the area of immunology has made great advances and clinical discoveries. However, it is necessary to develop methods to combat new diseases and strains whose resistance to antibiotics is growing more and more. For this, an in-depth investigation should be carried out on the functioning of the diseases, in order to give them a more rigorous microbiological approach. The ideas that are being studied are focused on the improvement of vaccines and the application of drugs such as sulphonamide to fight against diseases. Due to the rapid bacterial change and the lack of effectiveness in certain vaccines, it is necessary to carry out future studies that determine how to obtain more efficient vaccines and develop better anti-infective agents, as well as the improvement of their hosts27.
In the techniques of genetic editing important changes have been made over time, but it should be emphasized that the therapies are specific for each patient, thus being a huge challenge to improve the specificity and sensitivity of the techniques applied. The results that are expected in the future are improvements in both ex vivo and in vivo administration. In addition, in techniques such as CRISPR-Cas9 and DNA segmentation, important advances are expected in the treatment of poorly studied clinical diseases2.
Within monogenic disorders, the greatest limitation that has been found is not having an exact editing system, due to a large number of diseases derived from these disorders the studies required for each one have not been completed. The lack of studies reflects that much remains to be investigated, mainly on how to correct the lack of specificity in areas such as the correction of genes and editing by means of nucleases28.
Finally, the problems related to cardiovascular diseases are the lack of medical studies focused on collateral flow or metabolic improvements. In addition, approaches to the immunological responses of the treatments are required to obtain better clinical results. It is recommended that future clinical trials mix existing techniques such as bypass surgery or angioplasty therapies. Innovating and developing new methods of delivery or genetic editing are the fundamental steps so that patients who have potentially serious diseases have the possibility of accessing effective treatments that allow them to improve22.
CONCLUSIONS
Gene therapy has achieved great achievements in clinical trials to fight diseases. However, we must aspire to more rigorous processes that ensure a successful process for patients who are willing to try medical advances. Given that the impact of conventional and pharmacological treatments is not enough in certain diseases, the improvement of genetic editing represents an opportunity to carry out more specific processes in the future. Although the change in certain pathologies is constant, the innovation in genetic tools that are both in development and already existing would allow having more accurate and reliable results in the future.
REFERENCES
1. Ramamoorth M, Narvekar A. Biological K. Non Viral Vectors in Gene Therapy- An Overview. 2015;9(November 2013):1–7.
2. Maeder ML, Gersbach CA. Genome-editing technologies for gene and cell therapy. Mol Ther [Internet]. 2016;24(3):430–46. Available from: http://dx.doi.org/10.1038/mt.2016.10
3. Wirth T, Parker N, Ylä-Herttuala S. History of gene therapy. Gene [Internet]. 2013;525(2):162–9. Available from: http://dx.doi.org/10.1016/j.gene.2013.03.137
4. Ginn SL, Amaya AK, Alexander IE, Edelstein M, Abedi MR. Gene therapy clinical trials worldwide to 2017: An update. J Gene Med. 2018;20(5):1–16.
5. Collins M, Thrasher A. Gene therapy: Progress and predictions. Proc R Soc B Biol Sci. 2015;282(1821).
6. Husain SR, Han J, Au P, Shannon K, Puri RK. Gene therapy for cancer : regulatory considerations for approval. 2015;(June):554–63.
7. Wang D, Gao G. Technologies D. HHS Public Access. 2015;18(97):67–77.
8. Ding Q, Lee YK, Schaefer EAK, Peters DT, Veres A, Kim K, et al. A TALEN genome editing system for generating human stem cell-based disease models. Cell Stem Cell [Internet]. 2013;12(2):238–51. Available from: http://dx.doi.org/10.1016/j.stem.2012.11.011
9. Urnov FD, Rebar EJ, Holmes MC, Zhang HS, Gregory PD. Genome editing with engineered zinc finger nucleases. Nat Rev Genet [Internet]. 2010;11(9):636–46. Available from: http://dx.doi.org/10.1038/nrg2842
10. Wang H, La Russa M, Qi LS. CRISPR/Cas9 in Genome Editing and Beyond. Annu Rev Biochem [Internet]. 2016;85(1):227–64. Available from: http://www.annualreviews.org/doi/10.1146/annurev-biochem-060815-014607
11. Naldini L. Gene therapy returns to centre stage. 2015;1–10.
12. The Journal of Gen Medicine. Indications Addressed By Gene Therapy Clinical Trials [Internet]. John Wiley and Sons Ltd. 2018. Available from http://www.abedia.com/wiley/indications.php
13. Wang D, Gao G. Strategies T. HHS Public Access. 2015;18(98):151–61.
14. Prakash V, Moore M, Yáñez-Muñoz RJ. Current progress in therapeutic gene editing for monogenic diseases. Mol Ther. 2016;24(3):465–74.
15. Ghosh S, Thrasher AJ, Gaspar HB. Gene therapy for monogenic disorders of the bone marrow. Br J Haematol. 2015;171(2):155–70.
16. World Health Organization. Monogenic diseases [Internet]. Human Genomics in Global Health. Available from: https://www.who.int/genomics/public/geneticdiseases/en/index2.html
17. Cepika AM, Sato Y, Liu JMH, Uyeda MJ, Bacchetta R, Roncarolo MG. Tregopathies: Monogenic diseases resulting in regulatory T-cell deficiency. J Allergy Clin Immunol [Internet]. 2018;142(6):1679–95. Available from: https://doi.org/10.1016/j.jaci.2018.10.026
18. Koopman WJH, Willems PHGM, Smeitink JAM. Monogenic Mitochondrial Disorders. N Engl J Med. 2012;366:1132–41.
19. Xia E, Zhang Y, Cao H, Li J, Duan R, Hu J. TALEN-Mediated Gene Targeting for Cystic Fibrosis-Gene Therapy. Genes (Basel). 2019;10(1):39.
20. Hoernes M, Seger R, Reichenbach J. Modern management of primary B-cell immunodeficiencies. Pediatr Allergy Immunol. 2011;22(8):758–69
21. Rissanen TT, Ylä-Herttuala S. Current status of cardiovascular gene therapy. Mol Ther. 2007;15(7):1233–47.
22. Ylä-Herttuala S, Baker AH. Cardiovascular Gene Therapy: Past, Present, and Future. Mol Ther [Internet]. 2017;25(5):1095–106. Available from: http://dx.doi.org/10.1016/j.ymthe.2017.03.027
23. Lähteenvuo J, Ylä-Herttuala S. Advances and Challenges in Cardiovascular Gene Therapy. Hum Gene Ther. 2017;28.
24. German DM, Mitalipov S, Mishra A, Kaul S. Therapeutic Genome Editing in Cardiovascular Diseases. JACC Basic to Transl Sci. 2019;4(1):122–31.
25. Ylä-Herttuala S, Martin JF. Cardiovascular gene therapy. Cardiovasc gene Ther. 2000;355:737–51.
26. Singh S, Kumar R, Agrawal B. Adenoviral Vector-Based Vaccines and Gene Therapies: Current Status and Future Prospects. IntechOpen. 2016;i(tourism):13.
27. Casanova J-L, Abel L. The Genetic Theory of Infectious Diseases: A Brief History and Selected Illustrations. Annu Rev Genomics Hum Genet. 2013;14(1):215–43.
28. Cox DBT, Platt RJ, Zhang F. Therapeutic genome editing: Prospects and challenges. Nat Med [Internet]. 2015;21(2):121–31. Available from: http://dx.doi.org/10.1038/nm.3793
Received: 17 April 2019
Accepted: 23 May 2019
Nayade Sarmiento, Selena Tinoco
Genetic Engineering, School of Biological Sciences and Engineering,
YachayTech, Urcuquí. Ecuador.
Corresponding author: [email protected]